Agile Nations working group on fusion energy regulation: annexes to initial recommendations
Published 16 October 2023
Annex A: Fusion hazards and accident scenarios
A.1 Introduction
Unlike fission, fusion energy has inherent safety features, such as no risk of chain reaction or meltdown, fusion does not occur unless specific plasma conditions are met, and no high-level radioactive waste. It is important to consider regulations based on such characteristics related to fusion energy. Many of the globally proposed fusion approaches, however, do involve the use of tritium and the production of neutrons with related material activation hazards. Therefore, it is necessary to identify various events that are assumed as ‘worst-case scenarios’ and to evaluate the hazard and risk of each situation. This Annex provides 2 case studies on accident scenarios in fusion power plant, and the level of hazard in relation to potential public dose from the release of radioactive material.
A.2 Case study – UKAEA Technology Report
Fusion hazards
There are several different fusion technologies being developed, including magnetic confinement (eg. tokamak, stellarator), inertial confinement (eg. laser or projectile driven), or using a combination of both in magnetised target fusion. The majority will use deuterium-tritium (D-T) fuel and, therefore, the main radiological hazards for a fusion power plant will be tritium and the activated products created during operations. There are also other industrial hazards that need consideration, such as magnetic fields, lasers and hazardous materials, but existing regulations would be able to manage these hazards for a fusion power plant.
There have been several studies of potential accident scenarios based on evolving conceptual designs of future fusion power plant. Summary information and referenced literature on accident scenarios and analysis of fusion power plants can be found in the UKAEA Technology Report.[footnote 1] It is recognised that safety analyses are strongly dependent on the type of fusion technology, design of plant and the materials used. But the assessment of accident scenarios is dependent on the maximum inventory of radioactive material that could be released, so worst-case estimates can be used to identify the upper bounds of impacts.
Accident scenarios
A key safety feature of a fusion facility is the confinement of the radioactive substances at the facility (known as ‘inventories’): the tritium and activated materials described above. The multiple layers of protection provided by the confinement systems at the facility minimise the hazard of these substances to workers, the public and the environment. In an accident scenario, the confinement systems could be damaged, resulting in a percentage of these inventories being released into the environment that exceeds the annually permissible discharge levels set by regulators.
Based on a conceptual D-T tokamak-design fusion facility at power plant scale, UKAEA’s Fusion Safety Advisors have reviewed independent analysis, already published in the public domain, on the consequence of breaches of the multiple systems of confinement such as the vacuum vessel then the tokamak building. This exercise intended to illustrate the projected worst-case impacts to individuals that could arise from a release of the radioactive inventory from a fusion power plant. Supplementary information can be found in the UKAEA Technology Report.
This exercise identified 2 representative worst-case accident scenarios. The first Accident Scenario (Acc 1) is a breach of the primary confinement (eg. vacuum vessel) but with the tokamak building confinement and its filtration/detritiation systems intact. The potential worst-case dose is a few micro-Sieverts, which is similar to the radiation experienced on a single transatlantic flight. The second Accident Scenario (Acc 2) is a breach of the primary confinement, with an independent malfunction of the building filtration system, such that there is leakage through the tokamak building confinement. The potential worst-case dose is a few milli-Sieverts, which is similar to the radiation experienced from a CT scan of the chest.
An illustrative hypothetical ‘worst-case’ scenario (Hypo) resulting from an extreme magnitude earthquake to the facility was also considered. Dose consequence was estimated using an inventory-based approach whereby it was assumed that a large quantity of the tritium and radioactive dust inventory was released into the environment due to total failure of both the vacuum vessel and tokamak building confinement systems (although within the literature reviewed no sequence of events was identified that would lead to such total failure). The potential worst-case dose is less than a Sievert, above which there is potential for some acute radiation effects.
These accident and hypothetical scenarios[footnote 2] do not depict wholly realistic situations but serve to illustrate worsecase eventualities in order to identify the maximum potential hazard of a fusion power plant. By way of comparison, unmitigated or hypothetical accident scenarios in the aviation, oil and gas or nuclear fission industries could have similar or worse consequences that those described here for a fusion power plant, involving multiple fatalities and/or severe environmental damage. Worldwide, accident prevention and mitigations measures are put in place that considerably reduce the risks around such events. Such measures would be put in place for a fusion power plant.
Regulation of potential accidents
The Radiation (Emergency Preparedness and Public Information) Regulations 2019 (REPPIR 2019)[footnote 3] require facilities in Great Britain which handle specified quantities of certain radioactive materials to undertake emergency planning in proportion to the risks involved, considering both potential impact – in terms of dose to individuals – and likelihood. The way in which operators comply with REPPIR 2019 illustrates how UK regulation addresses hazard in a way that is proportional to that hazard.
The 2 indicative accident scenarios (Acc1 and Acc2) derived from publicly available research can be placed on the REPPIR 2019 Risk Framework matrix, illustrated in the figure below. The range indicated by the boxes take account of the uncertainties that remain in the technology and the fact that the scenarios described are based on conceptual designs, rather than detailed designs. The position of the hypothetical case (Hypo) is also indicated, however emergency planning would be based on the accident scenarios identified and assessed for individual designs.
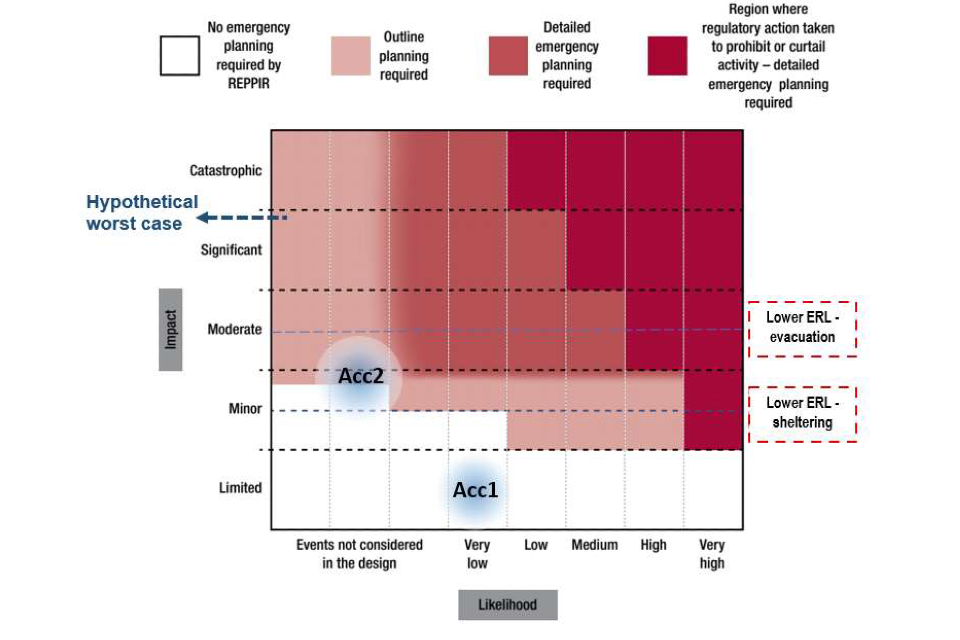
In summary, this analysis focused on the tokamak approach to fusion using the D-T reaction. However, it is expected that the findings from this analysis will be applicable to fusion power plant based on different technologies, such as inertial confinement and magnetised target fusion devices using D-T fuel. Although the detailed specifics of many of the accident sequences will be different, the worst-case radiological consequences are expected to be of the same order as those reported for similar accident scenarios.
Future analyses for safety and waste will be underpinned by greater technical certainty and information on inventory levels, which will reduce the uncertainty on the level of hazards. However, the hazards for fusion plant are well understood, and the continuing evolution of designs over the next couple of decades is unlikely to change the overall conclusions of this report.
A.3 Case study – JA DEMO
In order to grasp the safety characteristics of Japan’s DEMOnstration fusion reactor (JA DEMO), the Functional Failure Modes and Effects Analysis (FFMEA) has been carried out in National Institutes for Quantum Science and Technology (QST), where postulated initiating events (PIEs) have been selected and safety analysis regarding the PIEs has been performed. JA DEMO is currently designed as a pressurized water-cooled tokamak reactor with a major radius of ~ 8.5 m and a fusion power of 1.5 GW. The enthalpy of the cooling water of JA DEMO is about 5 times higher than that of ITER. Therefore, maintaining the soundness of the confinement barrier during In/Ex-Vessel Loss Of Coolant Accident (LOCA) is important from the viewpoint of ensuring the safety of JA DEMO.
As a result of the FFMEA, 14 events corresponding to system response accident events, and 5 events including superimposed events as the maximum PIEs were extracted. 13 out of 19 events were caused by the LOCA events. In these PIEs, the event with the greatest environmental impact was the guillotine break of the primary cooling pipe for the tritium breeding blanket as Ex-VV LOCA. In order to mitigate the pressurized effects against the Ex-VV LOCA, steam generators and heat exchangers with a large amount of coolant water retention is installed in the vault area, which has higher pressure tightness than other building areas, and a pressure suppression system (PSS) is connected to the vault area. The PSS connected to the vault also plays a role in passively recovering tritium contained in the water coolant vapor by the bubbling effect when the vapor containing tritium passes through during the Ex-VV LOCA.
For the worst-case of the Design Basis Accident (DBA) in the current design stage, which would be the event of the guillotine break of the primary cooling pipe, the use of the PSS against the Ex-VV LOCA brings a small public dose impact with a large safety margin to the evaluation target (< 5 mSv/event) at the site boundary (@ 1 km).
It should be noted that the maximum PIEs and the environmental impact will be revised when necessary according to the future design progress of JA DEMO. Furthermore, QST is considering the need for evaluation of Beyond DBA for the JA DEMO.
Annex B: Public perception
B.1 Introduction
Although there are differences in degree among countries, the current recognition and interest of fusion energy is relatively low. Government surveys, have provided some statistics around awareness and support for fusion energy amongst the public. In the aim of improving social acceptance of fusion energy, lessons can be learned from the nuclear fission industry. Strategic and long-term planning is important, including the promotion of appropriate initiatives and communications for each of the targets and priorities.
It is necessary to assure scientific safety, of course, but it is also necessary to be aware that people’s peace of mind is another matter. Thus consideration of public sentiment and scientific explanations for public understanding are also necessary. This Annex provides 2 case studies based on public surveys in relation to awareness of fusion.
B.2 Case study - Japan Government public acceptance and understanding activities
The government of Japan’s findings in relation to the public acceptance and understanding of activities regarding nuclear fission, recognise the challenges of how information should be provided to the public. The kinds of activities proposed to address these challenges can equally be applied to fusion.
It is necessary to respond to the public’s interest and strengthen efforts and activities by further promoting communication activities such as interactive dialogues and public hearings, while paying full attention to the uncertainties and risks of science. An information system based on scientifically accurate information and objective facts (evidence) must be established to promote an environment in which each citizen can form their own opinions based on a deeper understanding of such information. It is also necessary to respond flexibly to rapid changes in the means of obtaining information, including the Internet and social networking services (SNS), and to make constant improvements in the maintenance of information using various media.
The Atomic Energy Commission has proposed a structure[footnote 4] for stakeholder participation, considering the types and means of providing information, through to engagement and dialogue with the public:
In relation to public acceptance of fusion, each of these stages could be expected to take 3 to 4 years, and target public groups including school and university students, as well as stakeholders related to the industry. It would aim to build public trust by the following, conducting surveys at each step to gauge public attitude:
- establishing methods of dialogue with the public (including developing content and tools)
- creating a forum for dialogues with the public
- building social consensus with the public
In summary, regarding ‘fusion’, people still have a relatively low interest, and the public mood is not matured yet to discuss about the regulation of fusion energy. Thus public consensus needs to be made strategically step by step, considering the importance of inviting public opinion on the policy making process. Considerations include setting up a task force or working groups consisting members of the public alongside industry and regulatory specialists and science communication experts.
B.3 Case study - UK government Public Attitudes Tracker
The UK government Department for Energy Security and Net Zero (DESNZ) has a Public Attitudes Tracker, which since 2021 has included questions on Energy Infrastructure and Energy Sources. The survey includes questions related to fusion energy, and 4 sets of results have been obtained at 6 monthly intervals. The surveys are sent to a random sample of addresses, and are then completed on-line, with of the order of 4,000 to 5,000 respondents each time. The latest report[footnote 5] covers the surveys since 2021.
The questions aim to track the level of awareness and support for fusion energy, and includes the following background and questions:
Fusion energy is an experimental technology that works by fusing together atoms in order to release energy.
The UK is exploring whether this technology could be used to generate zero carbon electricity.
- Before today, how much, if anything, did you know about fusion energy ? (Figure 4.1).
- From what you know, or have heard about fusion energy, do you support or oppose the UK developing this technology ? (Figure 4.2).
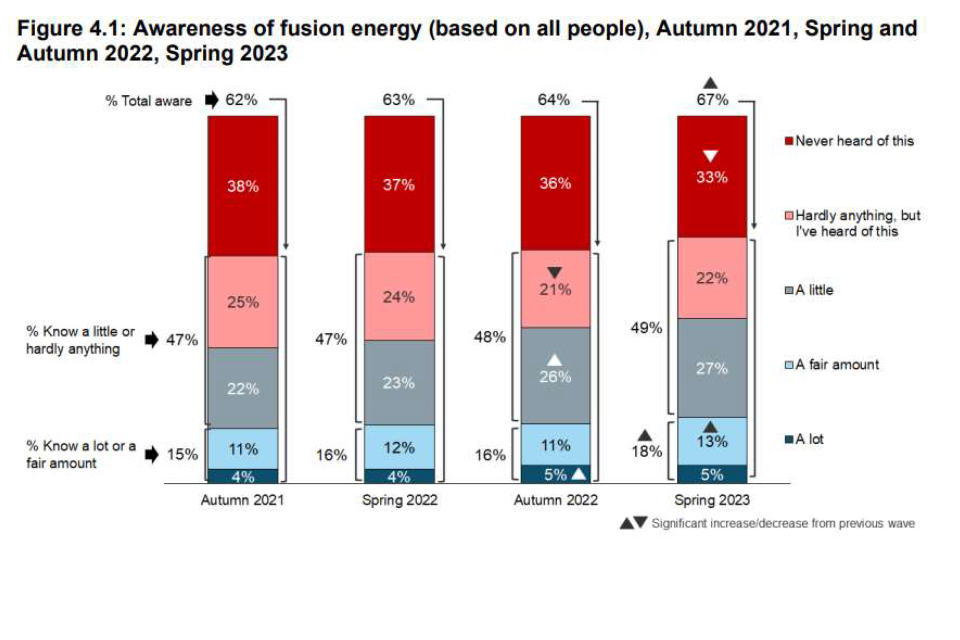
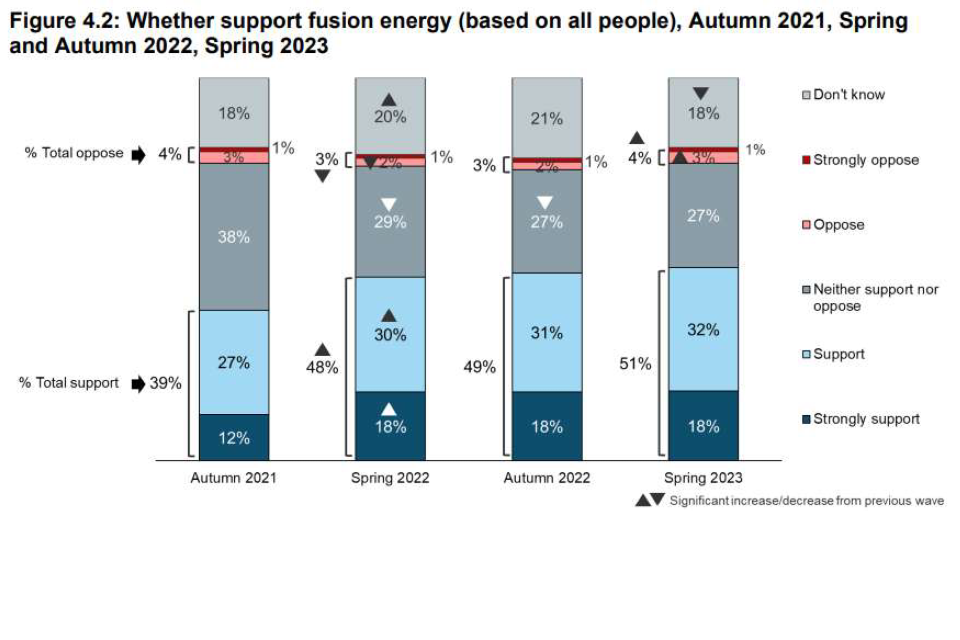
In summary of the public attitudes in the UK:
- Around half of those surveyed had not really heard of fusion energy, although awareness had increased by a few percent over the past couple of years
- Around half of those surveyed did not have a view either way on supporting or opposing fusion energy, reflecting the low overall levels of awareness
- Only 4% of those surveyed opposed development of fusion energy
-
Fusion Safety Authority, Technology Report – Safety and Waste Aspects for Fusion Power Plants, UKAEA-RE(21)01, Issue 1, 2021. ↩
-
Based on indicative radioactive inventory estimates relevant to a DT tokamak based fusion power plant. The information underpinning the accident scenario assumptions draws on studies of early conceptual designs. As the variables are strongly dependent on the technology, design and materials, as well as the accident scenario itself and any mitigations present, there is significant uncertainty around the maximum amount of inventory that could be released, but worst-case estimates used here seek to identify the upper bound. For all scenarios this analysis is based on worse-case assumptions in terms of estimated doses for the public at 1 km from the source. ↩
-
REPPIR 19 is the Radiation (Emergency Preparedness and Public Information) Regulations 2019. ↩
-
Provisional translation of Initiatives Related to Stakeholder Involvement, 2018, The Office of Atomic Energy Policy of the Cabinet Office - Japan. ↩
-
DESNZ Public Attitudes Tracker: Energy Infrastructure and Energy Sources, Spring 2023, UK. DESNZ PAT Spring 2023 Energy Infrastructure and Energy Sources ↩