Statement on the differential toxicity of particulate matter according to source or constituents: 2022
Updated 27 July 2022
Summary
1. Particulate matter (PM) refers to a minute portion of matter, frequently a very small solid or liquid particle (or droplet) of micrometre (μm) or nanometre (nm) dimensions. The most commonly employed metrics for measuring and regulating particulate matter air pollution in the environment are PM10 and PM2.5. The numbers 10 and 2.5 refer to the diameter in micrometres of the largest particles included in these categories. However, these metrics do not consider the sources or chemical makeup of PM.
2. Both epidemiological and toxicological studies have suggested that people’s health can be adversely affected by a number of chemical constituents and sources of PM. However, a 2015 COMEAP statement (COMEAP, 2015) concluded that the evidence at that time did not indicate that any one source or component is more strongly related with health effects than PM2.5.
3. Two comprehensive reviews on the topic (ANSES, 2019 and USEPA, 2019) have considered the evidence up to January 2018. In the light of these reviews, COMEAP has developed an updated statement based on these and other systematic reviews published until December 2020.
4. The literature reviewed highlighted that a number of different sources and constituents of PM are associated with adverse health outcomes across various exposure periods (‘short-term’ or ‘long-term’). PM (and PM constituents) associated with combustion and road traffic received the most attention, however, there was insufficient coherence in the epidemiological evidence to conclude that these sources of PM played a significantly greater role than other sources or constituents.
5. Overall, we conclude that, while the substantial body of recent evidence adds weight to the conclusion that different constituents are likely to have different toxicological actions, it does not consistently indicate specific components of PM that are more toxic than others.
6. While research assessing the chemical composition of PM should offer greater insights into toxicity than current metrics of PM, at present PM2.5 remains the most suitable metric for evaluating the health impacts, and hence for regulating PM in the atmosphere.
Background
7. Particulate matter is considered to be one the most harmful air pollutants, associated with high levels of mortality and morbidity. PM is often categorised in relation to its size range. PM10 and PM2.5 are PM with a diameter of less than or equal to 10 and 2.5µm, respectively. Coarse particles focus on the size range between 2.5 to 10µm and ultrafine particles (UFP) are particles with a diameter of less than 0.1µm. PM10 and PM2.5 are the most commonly employed metrics for epidemiological studies that look for associations with health outcomes, and for measuring and regulating PM pollution in the environment. Both metrics are mass based and do not consider the source contributions of chemical constituents of the particulate mixture.
8. Most airborne particles are internally mixed: there are multiple chemical components associated with each particle. This is a consequence both of the mixed nature of many emissions (for example, diesel particles are predominantly elemental carbon with many organic compounds, and brake wear particles contain many metal compounds co-emitted with an organic matrix), and of atmospheric processing by particle coagulation and vapour condensation onto particles. There are also multiple sources contributing to airborne PM, varying spatiotemporally. Thus, not only is there heterogeneity within single particles, but also there is chemical heterogeneity between particles.
9. This mixed nature has consequences for the lung as the primary receptor organ, the potential transmission of biological effects to systemic organs, and ultimately for the interpretation of epidemiological studies. Neither pure elemental carbon (Mills and others, 2011), nor secondary inorganics such as sulphate salts (Schlesinger and Cassee, 2003), show strong toxicity in human challenge studies, but both often show associations with adverse effects in epidemiological studies. These apparent contradictions are often explained by the component acting as a proxy for other more toxicologically relevant compounds co-emitted from the same source. For example, in the case of black carbon or elemental carbon (BC/EC), metals associated with fuel oil (vanadium, nickel and chromium) or organic constituents such as polycyclic aromatic hydrocarbons (PAHs), where there are established toxicological pathways. In the case of sulphate and its precursor gas, sulphur dioxide, it has been argued that the acidity of sulphate particles increases trace metal solubility and hence bioavailability increasing their toxicity (for example, COMEAP, 2009). Such explanations are plausible and there is a body of experimental evidence supporting both contentions, but at present these fall short of clear proof.
10. The BC/EC content of PM has been one of the most well studied constituents in relation to health-related effects (see, for example, WHO, 2012). Atkinson and others (2015) noted the relatively high coefficients associating EC with mortality in time-series epidemiological studies, but cautioned that the relationship may not be directly causal. One issue is that a component such as elemental carbon may comprise a relatively constant proportion of particle mass, and therefore be strongly correlated to particle mass. As a consequence, such a component may act as a surrogate for particle mass, and risks being incorrectly attributed the toxicity responsible for the adverse effects. It may also act as a carrier for other, more toxic constituents, as noted above.
11. While dependent on many factors (such as cohort, location, sources of PM, health parameter), a number of epidemiological studies attribute greater toxicity to PM2.5 particles than coarse PM, and give a mixed picture with respect to UFPs. This may result, in part, from the greater spatial uniformity of fine particle concentrations, leading to less exposure misclassification – exposure misclassification can bias results towards the null. Since chemical composition and particle size are often linked through particle production mechanisms, this may affect the ability of epidemiology to differentiate the effects of chemical components, especially when the PM2.5 size fraction is measured and there is a significant co-exposure to unmeasured coarse particles.
12. Much research has focused upon traffic-derived PM. The proportion of PM derived from non-exhaust emissions (NE-PM) is increasing due to the reduction in exhaust particle emissions following the fitting of diesel particle filters, and the increasing market penetration of battery-electric vehicles. The evidence for the toxicological and adverse health outcomes of NE-PM was reviewed by COMEAP in 2020 (COMEAP, 2020). The statement concluded that, while there was cellular and animal evidence that NE-PM has the capacity to induce toxicological effects (largely biomarkers of inflammation and oxidative stress in the lungs or blood), at present there was not sufficient evidence available to ascertain if NE-PM had adverse effects in real-world studies.
13. The COMEAP statement on the evidence for differential health effects of particulate matter according to source or components published in 2015 concluded that:
- it is unlikely that all components of particulate matter have the same potency in causing health effects
- there may be variations in toxicity between the various components of PM2.5, however, the evidence available did not give a consistent view of relative toxicity
- PM2.5 mass remained the preferred metric for quantitative assessments of the health effects of exposure to particulate air pollution
14. In coming to the views in its 2015 statement, COMEAP carried out a comprehensive assessment of the literature up to 2014. Notably, it emphasised the findings of 2 large-scale independent programmes of research that addressed PM composition and size: the World Health Organization (WHO) Review of Evidence on the Health Aspects of Air Pollution (REVIHAAP; WHO, 2013) and the Health Effects Institute (HEI) National Particle Component Toxicity (NPACT) Initiative (HEI, 2013). The overall conclusions of these research programmes informed those stated in the COMEAP 2015 statement.
15. Since then, 2 large comprehensive reviews have examined how the weight of evidence has changed in this area. A review by the French Agency for Food, Environment and Occupational Health and Safety (ANSES) entitled ‘Air pollution: new insights into ambient air particulate matter and the impact of road traffic’ (ANSES, 2019) commented on literature between 2013 and February 2016, while the US Environmental Protection Agency’s ‘Integrated science assessment for particulate matter’ (USEPA, 2019) considered evidence between 2009 to 2017. In the light of these reviews, and other systematic reviews published up to December 2020, COMEAP sought to provide an updated statement on the differential toxicity of components within PM. A small sub-group was established to undertake work on this topic, and its findings discussed by the full committee.
16. The particulate matter sources and constituents reviewed in this current statement broadly align with those of the ANSES (2019) and USEPA (2019) reviews. The main sizes of PM, PM constituents and sources of PM that were considered are:
- PM10 and PM2.5 (mass concentration)
- BC/EC-content, which is often used as an indicator of PM from combustion (for example from traffic exhaust, or in some countries from coal burning); this constituent of PM has been the subject of extensive research and was comprehensively reviewed by the WHO in 2012
- organic carbon (OC) constituents, including chemical groups frequently linked to toxicological actions such as PAHs
- metal content, particularly species that are redox active (for example iron, copper) or can exert effects such as inhibition of phosphatases and induce cellular kinase stress pathways: also, other metals that have been associated with health parameters or outcomes in epidemiological studies (for example, nickel, vanadium, zinc)
- oxidative potential (OP) of PM. An assortment of OP metrics, determined in purely chemical models, to assess the capacity of the pro-oxidant components of PM to drive damaging oxidation reactions in the human body
- nitrate and sulphate content of PM: the COMEAP (2015) statement noted these constituents as having “fairly consistent” associations with adverse health outcomes
- specific sources of interest such as traffic, coal or biomass burning, and so on, identified by source apportionment
- ultrafine particles of <100nm diameter
17. Some particulate properties were considered beyond the scope of this update of COMEAP’s statement (see Conclusions). These include:
- direct comparisons of PM2.5 with coarse PM (PM10 to PM2.5) or ultrafine PM (PM0.1, often measured using the surrogate of particle number concentration (PNC))
- specific sources of PM such as indoor PM, agriculture-derived PM, PM from domestic wood-burning, industrial sources of PM, and traffic NE-PM: we recognise the importance of these sources as a growing research need (see Research Recommendations). However, no systematic evaluations of the literature on these sources were revealed by the searches conducted, and a de novo synthesis of the finding of individual studies was beyond the remit of the Working Group
- occupational sources or exposures of PM, such as cooking environments, and exposures of garage workers, welders, underground transport workers, taxi drivers: due to the challenges of addressing source apportionment, attributing exposure to specific pollutants and the high number of confounding variables, occupational exposures to PM were considered beyond the scope of this report, as they were in most of the papers considered
- the biological constituents or contaminants of PM such as endotoxin content
- the consequences of any interaction between PM and gaseous co-pollutants
ANSES review
18. The ANSES reviewers took the REVIHAAP report (WHO, 2013) as their starting point, and considered subsequent literature up to February 2016. They addressed PM components (for example, EC and PAHs), particle size (coarse, fine and ultrafine) and sources (such as coal combustion, traffic, agriculture, and so on). Publications investigating PM10 and PM2.5 without chemical speciation or source apportionment were not included. Epidemiological (in humans) and toxicological (in animals) studies were considered, but not findings from in vitro or in silico studies (for example, cell cultures). Human ‘semi-experimental’ studies were included, for example, studies where students moved between urban and sub-urban areas, or in the vicinity of a steel plant, or cross-over trials with subjects travelling with or without air purifier respirators. Animal evidence was based on (sub)acute and (sub)chronic exposures, whereas single exposure studies alone were considered to be ‘inadequate’ levels of evidence. Health outcomes were separated into effects of short- (one to several days) and long-term (one to several years) exposures as well as further categorised as respiratory health, cardiovascular health, all-cause mortality, all-cause hospitalisations, neurological health, perinatal health, reproductive health, lung cancer, other cancers, and diabetes. The review aimed to address the quantity and quality of the evidence for individual constituents but not to rank the relative risks and potencies or toxicities of the various PM compounds or sources. In paragraphs 19 to 27, the sub-group has summarised the conclusions of the ANSES report.
19. There was a large volume of new studies to be considered, but the amount of evidence varied greatly for different PM constituents (see Figure 1). Associations were found for both/either short and/or long-term exposures, depending on the constituents and health parameter combination. Summary tables reflecting the weight of new evidence are replicated below.
20. Coarse and ultrafine PM There has been an increase in evidence supporting the potential for specific PM sizes to cause adverse health-related effects. While there was limited human evidence, the evidence for UFPs was largely from animal studies. From further human-based studies, coarse PM predominantly showed links with mortality (predominantly long-term exposures) and respiratory health (predominantly short-term exposures), whereas ultrafine PM was associated with cardiovascular (predominantly short-term) and neurological impairments following analysis of additional animal-based research strategies. The report recommends that further research should address the effects of these particle types on different organ systems.
21. Carbonaceous materials A large amount of evidence considered carbon species in PM, especially in human studies. BC/EC was linked to a variety of endpoints (for example, short- and long-term exposure was associated with all-cause mortality, respiratory disease and cardiovascular disease). From the limited evidence available for OC, both short-term and long-term exposure was associated with all-cause mortality and cardiovascular and respiratory conditions. There was insufficient evidence available for PAHs in PM (although these can be closely associated with EC and OC, for which there is more evidence available).
22. Transition metals A moderate amount of evidence found that certain metals in PM were associated with all-cause mortality (iron, short-term), and cardiovascular and respiratory disease (nickel and vanadium, short-term).
23. Secondary inorganic aerosols (Secondary aerosols are particles formed from gases and vapours within the atmosphere, normally by oxidation processes. Secondary inorganic aerosol comprises predominantly sulphates and nitrates formed from sulphur dioxide and oxides of nitrogen. Secondary organic aerosol is formed from the oxidation of volatile organic compounds to form less volatile oxygenated organic molecules.) Further evidence was found of associations between adverse health outcomes and nitrate- and sulphate-rich PM in epidemiological studies, but there was limited evidence for health-related effects from animal studies. The report notes that nitrates and sulphates may modulate the toxicity of PM rather than having an intrinsic toxicity themselves. There was little evidence found for ammonium ions and no conclusion was drawn on potential adverse health effects.
24. Road traffic Overall there were high levels of evidence linking traffic pollutants to adverse health parameters or outcomes. The highest levels of evidence in humans were for short-term exposure to traffic-related BC (mortality, respiratory and cardiovascular) and road dust (respiratory). A modest amount of new evidence from animal studies confirmed the capacity of vehicle exhaust to have detrimental biological actions. It was highlighted that the majority of these studies did not consider the potential role of nitrogen dioxide (NO2), a notable air pollutant from traffic, and there is potential that the effects of NO2 could influence the observed associations between traffic-derived PM and the health outcomes reported.
25. Coal combustion There was strong evidence confirming that short- and long-term exposure to emissions from coal combustion are linked to adverse health outcomes, particularly for household coal combustion in developing countries.
26. Non-anthropogenic sources From the limited studies available, there was moderate evidence to suggest that desert dust has adverse health effects, especially respiratory effects in children. In contrast, the evidence did not allow a firm conclusion to be drawn over whether sea salt and sea spray have adverse health effects, which could support the evidence of no health effect.
27. ANSES conclude that the evidence reviewed confirms the conclusions of the REVIHAAP report, in that a number of PM constituents and sources are linked to detrimental health-related effects. They recommend that as part of public policies to improve air quality, there should be priority given to targeting 3 currently unregulated particulate indicators: ultrafine particles (<100nm, particle number concentration), BC and OC. They also highlight “the need to act on the main controllable sources of emissions that include road traffic, coal combustion, petroleum product combustion and biomass combustion”. ANSES also highlights the lack of available evidence on the health effects of outdoor ambient air pollution from certain anthropogenic sources such as agriculture, maritime transport and airport traffic.
Figure 1. Overview of the conclusions reached by the ANSES report. Taken from ANSES (2019). Reproduced with permission of ANSES (as provided for by article L321-1 of the code of relations between the public and the administration)
1a. Categorisation by PM size, constituent or chemical property
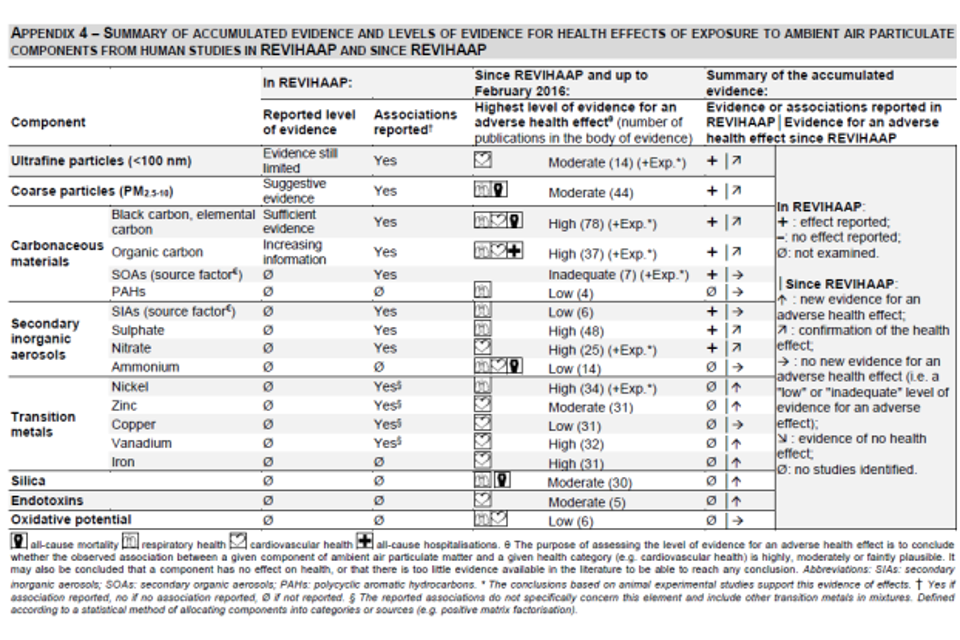
1b. Categorisation by PM source
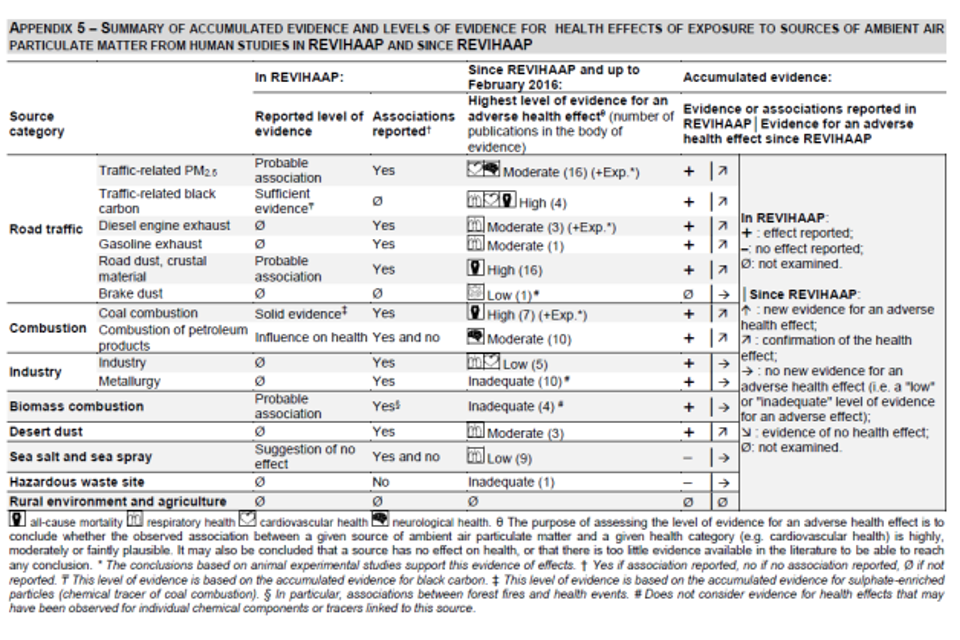
USEPA review
28. The September 2021 supplement to the 2019 ISA for PM (External Review Draft) reiterates the information on PM sources and components presented in the 2019 Integrated Science Assessment (ISA) for Particulate Matter. The USEPA (2019) considered the evidence for PM components (for example BC/EC, OC, nitrates, sulphates), particle size (coarse, fine, ultrafine) and sources (for example, source apportionment for industry, traffic, wildfires) published between January 2009 and January 2018. The report considered studies from countries with PM2.5 levels considered representative of the USA, such as Europe and Canada. Health outcomes were categorised as respiratory, cardiovascular, nervous system effects, reproductive and development effects, cancer and mortality. Discussion was separated into the different health categories, and conclusions were separated into effects of short-term (hours to approximately one month) and long-term (one month to years) exposures (summarised in table ES-1 of the report). The review also considered controlled exposures in humans (for example, chamber studies) whereby the PM effects could be separated from the rest of the pollution mixture (for example, by filtering out the particles from the mixture). Animal evidence was also considered, including both inhalation and instillation routes of pollutant delivery. In vitro studies were largely excluded, although studies that “provide mechanistic insight or examine similar effects as in vivo studies” were considered.
29. Mortality Positive Associations were found for many PM constituents but no one constituent generated substantially greater or stronger associations than another constituent. There was more evidence addressing components of PM rather than sources, although some positive associations were found for sources representative of traffic, coal combustion and biomass combustion.
30. Respiratory Short-term exposure to PM2.5 mass, sulphates, nitrates and BC/EC were associated with detrimental effects on respiratory health. BC/EC was the most well studied, although the evidence contained both positive associations and no-associations. There were fewer long-term studies, again predominantly on PM mass or BC/EC. Very few studies carried out source apportionment, although a few raised traffic and biomass combustion as important sources.
31. Cardiovascular The evidence for cardiovascular endpoints was broadly similar to that of the respiratory outcomes. Short-term and long-term studies showed mostly positive associations. Many considered BC/EC, although it was not possible to conclude if BC effects were independent of PM2.5 mass. Of the limited source apportionment studies, links were found for combustion activities such as industry, traffic and wildfires.
32. Assessment of the causality of associations found that for mortality, respiratory and cardiovascular outcomes there was broadly a ‘likely causal’ relationship for PM2.5. For coarse PM, the accumulated evidence was “suggestive” of causality for various health outcomes, whereas there was only limited new evidence to assess the causality of relationships for ultrafine PM. There was only suggestive or insufficient evidence for the other health outcome categories. A summary table of the change in evidence and causality assessment is replicated in Figure 2.
33. Overall, it is concluded that “recent studies continue to demonstrate that many PM2.5 components and sources are associated with both sub-clinical and clinical endpoints (for example, hospital admission and mortality)”. However, “the evidence does not indicate that any one source or component is consistently more strongly related to health effects than PM2.5 mass.”
Figure 2. Overview of the conclusions reached by the USEPA report. Figure taken from USEPA’s Integrated Science Assessment (ISA) for Particulate Matter (USEPA, 2019)
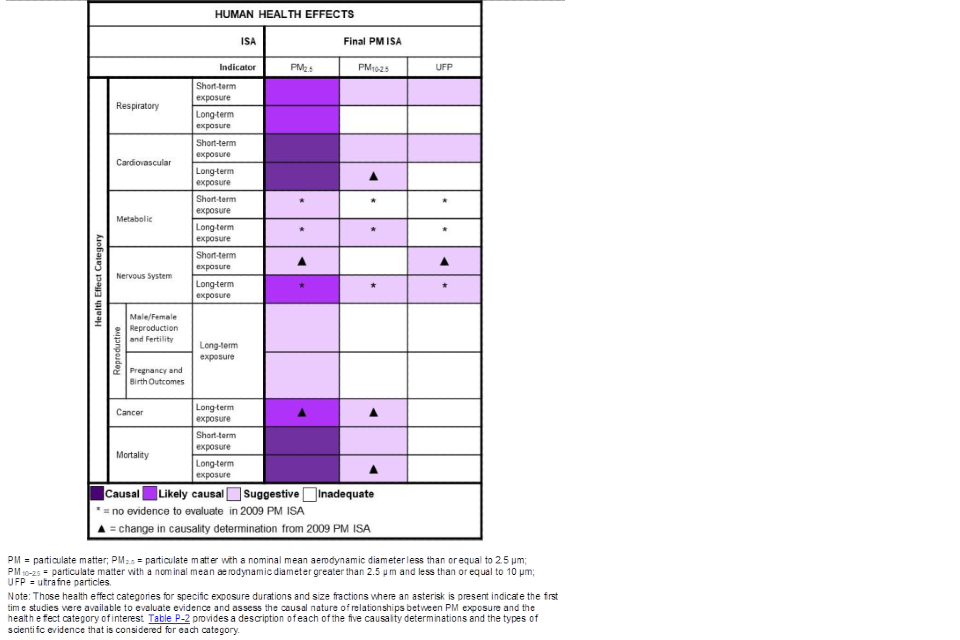
Other documents considered
34. Given that the ANSES review considered evidence up to February 2016 and the USEPA until January 2018, the COMEAP sub-group also considered more recent evidence. A review of the individual original research articles was beyond the scope of the current report, therefore, the sub-group sought to find other comprehensive systematic reviews published between January 2016 and December 2020. Reviews were identified by the members’ own knowledge and a literature search to identify other systematic reviews on this topic. Seven reviews were identified as being potentially relevant (discussed below). A number of specific research papers addressing a relevant aspect were also reviewed.
Rohr and McDonald (2016)
35. This is a detailed, but not exhaustive, review of the sources, properties and health-related effects of carbonaceous particles, that is those containing elemental carbon and organic compounds. It is not a systematic review and contains no meta-analysis. It first considers the sources and properties of carbon-containing particles in the following categories: engine emissions, wood or biomass combustion emissions, biogenic emissions and secondary organic aerosol (SOA), resuspended road dust, tyre and brake wear, as well as cooking emissions. This is followed by a review of epidemiological and toxicological studies addressing each category of particles, and a summary of recent large toxicological and epidemiological research programmes, including SPHERES, NPACT, NERC, ACES, and TERESA.
36. Its overall findings are neatly summarised as: “a review of the extant literature suggests that there are clear health impacts from emissions containing carbon-containing PM, but difficulty remains in apportioning responses to certain groupings of carbonaceous materials, such as organic and elemental carbon, condensed and gas phases, and primary and secondary material. More focused epidemiological and toxicological studies, including increased characterization of organic materials (specifically PAH speciation), would increase understanding of this issue.”
Achilleos and others (2017)
37. This is a systematic review and meta-analysis of studies published up to July 2015 which linked short-term PM2.5 exposures with all cause, cardiovascular and respiratory mortality.
38. A specific focus was upon linking risk estimates with city-specific characteristics, with a view to explaining the heterogeneity of risk estimates, but little consistency was found. From studies reporting measurements of specific particle components, for most constituents there was a high variability in effect estimates between cities. When PM2.5 mass was controlled for, associations were found between EC and potassium with all-cause mortality, and between vanadium and cardiovascular and respiratory mortality. The strongest and most consistent associations were with EC and potassium (normally a marker of biomass combustion), suggesting the importance of combustion sources, albeit probably of different fuel types and overall chemical composition. The authors also highlighted the important influence of measurement error, which tends to bias the results towards the null for the less accurately measured species, which would include high spatial variability as a cause of error.
Ohlwein and others (2019)
39. This is a systematic review of epidemiological studies published between 2011 and 2017 investigating possible health effects of ambient UFPs. A total of 85 original studies were identified, with the vast majority (n=75) being investigations of short-term exposures: mostly panel studies, time-series, case-crossover studies or studies in which participants were exposed in pre-defined settings (such as commuting by bike).
40. In most studies, UFP were assessed as particle number concentration (PNC) per volume of air, only a few studies measured particle mass or lung-deposited surface area. For PNC, lower cut points ranged between 3nm and 250nm. Only one-third of the studies investigated particles below 100nm, which is the normally adopted upper limit for UFP sizes. Hence, the review includes many studies which do not meet the normal definition for UFP, and in some cases appear not to have included UFP.
41. Panel (n = 32), scripted exposure with predefined settings (n = 16) or time series studies (n = 11) were most frequent. Other pollutants (including PM2.5, NO2, ozone (O3) and sulphur dioxide (SO2), although which pollutants investigated varied across the studies) were assessed in 78 studies, but only 34 studies adjusted for co-pollutants in the statistical analysis.
42. The most consistent associations were identified for short-term effects on pulmonary or systemic inflammation, heart rate variability and blood pressure, that are independent of other pollutants. For other health outcomes, the evidence was insufficient or inconclusive.
43. The authors suggest that a future challenge is to develop enhanced, thoroughly validated, spatiotemporal models which can contribute to a more precise exposure assessment across larger areas (important given the relatively high degree of spatiotemporal variability in UFP concentration) as well as incorporating multi-pollutant models to elucidate the independence of effects.
Yang and others (2019)
44. This study is a meta-analysis of studies before August 2018, considering 42 studies in the qualitative assessment and 35 in the quantitative assessment. PM2.5 constituents include BC/EC, OC, nitrate, sulphate, ammonium (NH104+) and selected metals. Coarse PM was not included. Sources of PM were considered in the review, but there were too few studies to draw conclusions. Results are presented as percentage excess risk (ER) per interquartile range (IQR) of each constituent.
45. Overall, the most robust associations with non-accidental mortality were found with BC (ER=0.33% per IQR), OC (ER=0.35% per IQR) and potassium (ER=0.37% per IQR) in the short-term studies. Long-term associations with non-accidental mortality were found for BC (ER=1.78% per IQR), nitrate (ER=2.6% per IQR), zinc (ER=9.4% per IQR) and silicon (ER=5.90% per IQR). The very large coefficients for long-term exposure to zinc and silicon are based on a very small number of studies (n=2), and the short-term exposure studies for these constituents showed only very small effects. Cardiovascular mortality and morbidity (hospital admission) were associated with BC, OC and nitrate. Short-term respiratory outcomes were associated with OC.
46. Other ‘potentially harmful’ constituents were nitrate, sulphate and vanadium for respiratory outcomes, and nitrate, sulphate, zinc, silicon, iron, nickel, vanadium and potassium with adverse cardiovascular outcomes.
47. The investigators conclude that their analysis “suggests that black carbon and organic carbon are important detrimental components of PM2.5, while other constituents are probably hazardous to human health”. The narrative of the analysis is very concise, and it is not clear if the conclusion is largely based on the number of positive associations found for BC and OC, or other factors such as the magnitude of effect, significance after adjustment for confounders or interpretation of these results with other studies (we assume all of these factors but perhaps a greater emphasis on the former). Additionally, within each category, the maximum number of studies was 4 or less, so the confidence in these findings is limited.
Cassee and others (2019)
48. This ‘White Paper’ is a report arising from a meeting on ultrafine particles that was attended by experts in both ‘exposure’ and ‘toxicology’ of ultrafine particles. It should be highlighted that the term ‘ultrafine particles’ in the report is often generalised to include manufactured or nanoparticles produced within occupational settings, as well as ultrafine particles in ambient air. The report is not a systematic review of the literature. However, a number of views in the report are worth highlighting below.
49. The report notes that “focusing only on PM2.5 may result in overlooking the impact of UFP”.
50. The high spatial and temporal variability of UFPs presents a problem for epidemiological studies even if better UFP monitoring and modelling could be established. Nonetheless, recent epidemiological studies (Downward and others (2018), Bai and others (2019)) attempting to address the challenges of spatial distribution suggest UFPs may have effects on cardiovascular morbidity that are independent of PM2.5 and NO2.
51. “While shorter averaging times than 24 hours seem relevant to determine the health impact of UFP, there is a lack of data on long-term exposure to UFP from experimental studies.”
52. The authors suggest that the toxicological evidence is more consistent in regard to UFPs having a greater potential to induce harm than larger particles. “The toxic potency of UFP when using mass as a dose descriptor differs from the PM2.5, (but not always) showing that UFP cause greater effect. Moreover, the lung shows a different response to UFP compared to larger particles.”
53. Furthermore, “it is clear that UFP collected from different locations (and sources) possess different toxic potencies” and “there are considerable differences in the toxic potency of UFP from various sources when using mass as a unifying metric. Soluble components, including organic chemicals, appear to contribute to the effects of UFP, but the key drivers of differences in toxicity remain to be determined.”
54. One notable conclusion of the report is that “while the health effects of UFP have been substantiated based on toxicological evidence, there is a need to systematically and quantitatively assess the existing evidence based on epidemiological research.”
55. In relation to monitoring, “due to the lack of instrumental methods we cannot recommend UFP mass or surface area concentration measurements as routine approaches”, although “the common air quality monitoring strategy (at least in the EU) should be extended by set-up of so called ‘supersites’ … with size-segregated UFP measurements and online BC.” However, the report notes that the challenges of accurate monitoring of UFPs in the environment represent a considerable barrier to their regulation.
Kelly and Fussell (2020)
56. This review describes the complexity of understanding the differential toxicity of PM. However, it is not intended to be a comprehensive analysis of the recent literature in this area, but instead to be an overview and commentary of the topic addressing knowledge gaps.
57. Of note, the review highlights 2 types of PM that require further investigation: non-exhaust traffic-derived particulate matter (NE-PM; brake, tyre and road wear) and micro-or nano-plastic PM. This research is required given the limited toxicological and epidemiological information on these types of particles despite the potential for high levels of exposure to the public. The review also highlights the need for cell, animal and human controlled exposure studies to directly compare the actions of different PM on health parameters within the same study and the use of global integrated omics expression approaches.
Weisenberg and others (2020)
58. This paper provides a narrative review of data from 4 specific geographical locations, encompassing both spatial and temporal examples: Bitterfeld (Germany), Utah Valley (USA), before, during and after the Olympic games in Beijing (China) and Viadana (Italy). Analysis of these areas was focused upon a defined literature search from which environmental pollution characteristics (for instance PM level, heavy metal and NOx levels) were extracted, alongside health outcomes that included prevalence of asthma, lung function, cardiovascular health, skin disease, as well as hospital admissions or school absence. Experimental studies using air pollutants from several of these sites, relating to potential human health effects, were also observed from the literature.
59. As an example of the spatial comparisons, in the Bitterfeld Study there were contrasting levels of PM, heavy metals and nitrogen oxides (NOx) between the cities of Hettstedt and Bitterfeld due to differences in local industrial processes. Increased prevalence of asthma, hay fever and atopic dermatitis were found in the city sites compared to a rural setting (Zerbst). Experimental studies in humans and animals found that PM2.5 from Hettstedt had greater inflammatory and pro-allergenic effects than PM2.5 from Zerbst. Weisenberg and others suggested that the higher metal content of PM from Hettstedt led to it being more detrimental to health. Studies on the other spatial example, Viadana, concerned children living near 2 chipboard factories in Northern Italy, but focused on gaseous pollutants (formaldehyde and NO2) rather than PM.
60. As an example of the temporal comparisons, the authors consider data from before, during and after the Beijing Olympic Games. Levels of PM, heavy metals and NOx dropped during the games, and increased again afterward. The changes in pollutant levels were associated with differences in cardiovascular and lung parameters in adults, and birthweight. In the other temporal comparison, reductions in respiratory ill-health (bronchitis), school absences and hospital admissions were reported during a period when a steel mill in Utah valley was closed, and pollution levels lowered.
61. The paper highlights that varying levels of PM from different sources and different composition can impact negatively upon human health, but does not reach firm conclusions in regards to which sources or constituents drive the various changes in health parameters or outcomes.
Oxidative potential of PM
62. A search of epidemiological studies using OP as an exposure metric revealed 22 studies (see Annex A), the majority of which (14) were panel studies using biomarkers of respiratory or cardiovascular effects as health end points. There were also cohort studies of longer-term exposures (4) and time series or case-crossover studies of acute effects (4) considering outcomes as diverse as low birth weight and mortality. The OP metrics were also diverse, including the dithiothreitol (DTT) depletion, glutathione (GSH) or ascorbate oxidation, and electron spin resonance (ESR/EPR) in vitro assays and cellular reactive oxygen species (ROS) generation assays. Most studies used a range of exposure variables, in some cases including particle mass, while others used only OP or included chemical constituents, but not mass.
63. The overall picture is very mixed, with some studies showing an OP metric to be a better predictor of the health outcome than mass, some which conclude that mass is the better predictor, and some in which OP is concluded to be an effect modifier of outcomes attributed to mass. Overall, given the large range of study designs, health endpoints and the variety of OP metrics, it is perhaps not surprising that the conclusions as to the suitability of OP as an exposure metric for predicting adverse health outcomes are also highly diverse. One notable feature, no doubt reflective of the fact that the various OP tests respond most strongly to different components of the particles, is that in studies where OP was an effective predictor of health outcomes, it was often the case that one OP test and not another was associated significantly with a health outcome.
64. Toxicological research has also utilised OP measurements of PM, alongside other detailed chemical analyses, to assess the potential toxicity of different sources and components (Kelly and Fussell, 2015). For example, the OP of ambient PM per unit mass collected at busy roadside sites is clearly enhanced relative to urban background and rural sites, which appears to be attributable to enrichment of metals linked with abrasion processes, and not to particle size (Godri and others, 2011). The OP of combustion-related PM is found to be more than 2 times greater than that of Saharan dust outbreak samples, indicating that the source of PM OP and PM mass are not the same. The OP of particles emitted during the burning of mixed biomass and wood is considerable (and greater than that of diesel exhaust, gasoline exhaust and residual oil fly ash particles). Bioaerosols, specifically fungal spores, exhibit OP levels similar to those of metals and quinones. The type of OP assay used can generate different, even contrasting, relative OP signals across a panel of PM types (Øvrevik, 2019). While this complicates the interpretation of the data, this also highlights that individual assays will preferentially detect the redox activities of the different PM constituents. Nonetheless, a number of studies have successfully used PM OP metrics to implicate particle redox properties as a key contributor to their toxicity, for instance their capacity to generate intracellular oxidative stress, mitochondrial dysfunction, and inflammatory pathways (Kelly and Fussell 2015).
Summary of the available evidence
65. The sub-group noted that a large volume of research on the differential toxicity of PM has amassed in recent years This literature has been comprehensively reviewed by the ANSES and USEPA reviews as well as a number of more recent systematic reviews. In many cases the detail and quantity of available evidence was emphasised rather than a rigorous critical appraisal of the different strands of evidence across studies.
66. Across the reviews there is agreement that different sizes and composition of PM will likely have different degrees of toxicity on different organ systems of the body through a range of different biological pathways. However, the amassed recent research does not provide further consensus as to which sources and constituents of PM are driving toxicological actions. Based on the evidence reviewed, we summarise our conclusions by constituent or source:
67. Black carbon/elemental carbon BC/EC was the most frequently studied constituent in the evidence that the sub-group assessed. BC/EC is often an important component of PM and has shown strong associations with adverse health outcomes in many studies. It can be regarded as a marker of combustion emissions, but as such represents a wide variety of sources, including both fossil fuel and biomass combustion, and consequently has a wide range of chemical composition and particle sizes. In many countries, BC/EC is a good tracer of diesel traffic emissions, but this is not universally the case. It is unclear whether the association with health outcomes is causal, and if so whether the BC/EC directly contributes to toxicity, or is instead due to the organic and/or inorganic components associated with it.
68. Organic carbon constituents While BC/EC content of PM has received the most attention, the term ‘organic carbon’ includes a huge number of both primary emissions and secondary components of particles which are unlikely to be of equal toxicity. The composition varies substantially from place to place, and may explain some of the heterogeneity in coefficients. Recent studies have attributed particular significance to the secondary component which is typically highly oxidised and may contain highly reactive species such as peroxides, but causal links to health have yet to be established. The evidence reviewed here did not address the toxicity of individual categories of organic carbon constituents, but equally it did not call into question the existing evidence that specific constituents, such as PAHs, have a well-established toxic potential.
69. Transition metals Toxicological studies, and especially some measures of oxidative potential of PM, suggest an important role for transition metals in the toxicity of airborne particles. However, there is a paucity of evidence from epidemiological studies, and that which is available does not give strong support to a major role for transition metals, which are generally minor components of PM by mass. There is some, limited evidence of associations of adverse effects with a number of metals including iron, zinc, nickel and vanadium, and such elements are known to influence oxidative potential tests. Some studies have attributed importance to potassium, which is not a transition metal, but can serve as a marker for biomass combustion particles.
70. Nitrate and sulphate Interest in inorganic constituents such as nitrate and sulphate content appears to have waned somewhat over the last few years. However, there is considerable support from epidemiological studies for a major role of sulphate and nitrate in PM toxicity, although this is not borne out by toxicological studies in humans or animals (Schlesinger and Cassee, 2003; Park and others, 2018). The suggestion that sulphate, in particular, is associated with other, more toxic, trace constituents, or acts to solubilise trace metals may offer an explanation.
71. Oxidative potential of PM Oxidative stress is believed to play an instrumental role in the capacity of particulate pollution to cause cell and tissue damage. The oxidative potential of the PM (typically in acellular assays) has been used as an effective exposure metric, identifying toxic components and sources within diverse ambient PM mixes that populations are subjected to, from traffic emissions on busy roads in urban areas, to biomass smoke that fills kitchens in rural areas of the developing world. However, the application of these metrics in epidemiological studies is very inconsistent due to diversity of the techniques used to measure this parameter and the frequently poor correlation of different measures of OP which are driven by different components of the particles. Additionally, the sources of PM mass and sources of PM oxidative potential are not necessarily the same, therefore targeting mass would not necessarily have equivalent mitigatory effects on PM OP. Finally, OP metrics derived in acellular models will only reveal their inherent capacity to drive damaging oxidation reactions. In vivo, 2 things need to be considered: first, that cells and tissues will respond adaptively to the imposition of oxidative stress, and second, that there are many PM constituents that, although not redox active, will generate cellular ROS generation either through the stimulation of cellular enzymes, as a function of their metabolism, or by induction of inflammation.
72. Coarse particles There is far less evidence on the effects of coarse, than of fine, particles (PM2.5). That which is available suggests that toxicity extends into the coarse particle size range, but epidemiological studies may underestimate the magnitude of effect due to much greater exposure misclassification than in studies of fine particles due to lower spatial uniformity of concentrations.
73. Ultrafine particles Toxicological evidence strongly supports the suggestion that UFPs have a greater capacity to induce adverse (and possibly different) biological effects than their larger particle counterparts from similar sources. However, at present the epidemiological evidence is highly inconsistent in regards to whether metrics of the ultrafine fraction of PM2.5 are more strongly associated with adverse health outcomes than total PM2.5. There are many reasons for this inconsistency: the lack of monitoring of UFP, the high number of sources of UFPs in outdoor and indoor environments (and that the ultrafine fraction may in itself originate from different sources than the larger particles in PM) and the high spatial and temporal variability of UFPs. Specific sources of PM such as diesel exhaust are rich in ultrafine particles. These particles agglomerate after emission and so are not entirely in the ultrafine range when airborne. Nonetheless the ultrafine fraction predominates (for example, in controlled conditions, more than 85% of the particle numbers in diesel exhaust were ultrafine (Mills and others, 2011)), and larger particulates may de-agglomerate into smaller particles in the lung lining fluid and other biological compartments. It has already been established that diesel exhaust can induce lung inflammation and adverse effects on the cardiovascular system in human controlled exposure studies, however, this approach has not been used to ascertain the biological effects of lower exposure regimes or directly compare different sources, constituents or sizes of PM. Sources such as those from vehicle exhaust, may contain a number of harmful constituents (for example organic carbon compounds, constituents with high oxidative capacity) that could increase their potential toxicity. UFPs also have a greater surface area per unit mass, which some studies have suggested as important in driving toxicity, alongside UFP surface reactivity and number. It is also prudent to note that a proportion of UFPs can reach areas of the body that larger particles (that is, fine particles) are unable to access.
74. Specific sources A small number of studies have conducted source apportionment of PM by receptor modelling prior to epidemiological analysis in order to associate health outcomes with specific sources of particles. The results show little consistency, but this may arise from the large combined uncertainty of receptor models and epidemiological models, and the often-limited size of data sets for epidemiology due to the large input data collection required by receptor modelling. With the exception of traffic and combustion sources (for example, coal and biomass combustion) there were relatively few studies that link together the source, associated constituents and their toxicological or adverse health outcomes. While there is a lack of coherence in the findings from epidemiological studies, the toxicological profile of combustion derived particles (especially combustion-derived UFPs from vehicles) suggests that this source of PM could be associated with detrimental biological effects beyond that of PM mass concentration per se. Furthermore, these pollutants are ubiquitous in many environments, especially urban environments with a high population density. (Since the completion of our review, the Health Effects Institute (HEI) has published (June, 2022) a systematic review of health effects of long-term exposure to traffic-related air pollution, which found evidence for associations with a range of adverse health outcomes, including with EC and traffic-derived PM2.5.) There is also some evidence from source-specific studies of toxicity associated with particles emitted from biomass burning.
75. Desert dust, silica and volcanic ash Epidemiological studies on desert dust have examined short-term exposures by comparing ‘dust’ and ‘non-dust’ days. Reviews of the available evidence indicate an effect on cardiovascular mortality and respiratory morbidity, although some inconsistency in the results of studies and across regions has been noted. Studies of long-term exposure are lacking (WHO, 2021). Experimental research on desert dust provides support for biological plausibility of epidemiological associations between this particulate air pollutant and events including exacerbation of asthma, hospitalisation for respiratory infections and seasonal allergic rhinitis (Fussell and Kelly, 2021). Studies, in the main using doses that reflect or at least approach real world exposures during a dust event, have demonstrated that virgin sand dust particles and dust storm particles sampled at remote locations away from the source (and as such, laced with industrial pollutants and micro-organisms) induce inflammatory lung injury and aggravate allergen-induced tissue injury. Whilst comparisons with urban particulate matter less than 2.5μm in diameter (PM) suggest that allergic inflammatory responses are greater in microbial element-rich dust-PM2.5, aerosols generated during dust events appear to have a lower oxidative potential compared to combustion-generated PM2.5 sampled during non-dust periods. A recent review by Aghababaeian and others (2021) summarised this literature and reached similar conclusions. Quartz silica in volcanic ash has also been shown to have toxicological actions, although this was not explored in any depth in literature that the sub-group assessed.
76. Sea salt Sea salt is considered to be a relatively inert constituent within PM. A study in Barcelona, Spain (Ostro and others, 2011) performing source apportionment did not find associations with ‘aged sea salt’ and all-cause or cardiovascular mortality. In a human controlled exposure study, concentrated ambient particles that were composed of 30 to 90% salt were found to have no effect on cardiovascular parameters that are impaired by similar exposures to diesel exhaust (Mills and others, 2008). However, there are very few studies which have looked explicitly at the effects of sea salt, and insufficient to draw firm conclusions, and it remains possible that they are non-toxic. It should be borne in mind that although sodium and chloride are the main constituents, sea salt is a complex mixture of inorganic salts with trace elements present, and contains organic matter which is enriched during the formation of marine aerosol. It also ages rapidly in polluted atmospheres leading to changes in composition, which may increase its ability to induce adverse effects. While sea salt may be one of the sources or constituents with a lesser toxicity than others, the sub-group’s view is that there is insufficient evidence to exclude any component of PM2.5 as being health-relevant.
Causality
77. The 9 guidelines proposed by Hill (1965) are commonly applied as criteria when evaluating the likelihood of the causality of associations observed in epidemiological studies. While it is generally accepted that the effects attributed to PM2.5 exposure meet the requirements of most of the criteria, and hence suggest that associations are causal, such a comparison in relation to specific particle components raises major problems. In particular, the criteria of consistency between studies and especially of coherence between laboratory and epidemiological studies are not well satisfied for any specific component or source of airborne particles.
Conclusions
78. In its 2015 evaluation of this topic, COMEAP expressed the view that:
“Particulate air pollution is a complex mixture of many chemical components. Although it might be expected that some components are more harmful to health than others, the evidence available from population-based studies does not give a consistent view of their relative toxicity. Both particles emitted directly from a range of pollution sources, such as traffic and solid fuel combustion, and those formed by chemical reactions in the atmosphere are associated with adverse effects on health and the current consensus is that these associations are, at least in part, causal. Hence, reductions in concentrations of both types of particles are likely to benefit public health.”
79. While the available evidence has expanded, and confidence in the causal effects of particulate matter upon health over a very wide range of concentrations has increased (COMEAP, 2021; WHO, 2021), the latest review of evidence, presented here, has not changed our view on the issue of differential toxicity of particles and their components. At present, the most robust relationships are between adverse health outcomes and exposure to the PM2.5 mass size fraction, and in our view the continued monitoring and regulation of PM2.5 is the best approach to the protection of public health. The measurement of specific components of particles is also encouraged as these have great value in research into particle dynamics and toxicity, and in source apportionment, and is hence of value in air quality management.
Research recommendations
80. While these recommendations are organised into broad categories, there are many overlaps across these categories that highlight the need for a multidisciplinary approach to determine the potential health effects of different constituents and sizes of PM.
Epidemiological methods
81. Additional large-scale epidemiological studies incorporating high quality source apportionment by receptor modelling or dispersion modelling are warranted, especially in relation to less well-studied sources of PM (see below). Studies of the effect of temporal changes in particle composition upon exposure-response coefficients in epidemiological studies may yield valuable insights. Innovative designs of epidemiological study capable of distinguishing the effects of correlated exposures are strongly encouraged. As different particle components appear to be linked to different mechanistic pathways, the study of health outcomes other than all-cause mortality in conjunction with particle speciation may provide useful insights into effects of differing particle types.
82. Also, there is a need to evaluate the relationship between pollutant exposures and early markers of disease in longitudinal cohorts in order to address the contribution of air pollution to the development of non-communicable diseases.
83. Further clarity may be obtained from systematic reviews and meta-analyses of studies that make comparisons between specific PM sizes or constituents and health-related outcomes, for example. PM10 and PM2.5, PM2.5 and UFP metrics (such as particle number concentration) or PM2.5 and BC/EC.
84. In recognition of the high relative surface area of UFPs, and increasing evidence that these particles gain access to the blood and systemic organs, these particles warrant continued attention. In particular more research is required on the source and composition of UFPs, exposure levels and how exposure to these may impact on different organ systems and, accordingly, population groups with different risk factors and health conditions.
85. More research is needed on sources of PM for which the potential effects on health are less well studied, including indoor PM, non-exhaust traffic-derived PM, micro- or nano-plastics, PM from burning wood, PM-derived from agricultural emissions, and PM from shipping and aviation. The need for research into the health-related effects of these emissions aligns with the UK Clean Air Strategy (2019). The risks associated with PM from wildfires also represents a pressing research need given the increasing prevalence of wildfire events internationally. Toxicological studies directly comparing PM from these sources to that of more established sources would be beneficial (for example, Selley and others, 2020).
Monitoring needs
86. Monitoring strategies for airborne particles should account for the needs of different types of epidemiological study, including factors such as temporal and spatial variation of particle components, and relationships between outdoor and indoor microenvironment concentrations. One of the main limitations on research in the UK is the paucity of consistent long-term data sets of speciated chemical composition for airborne particles, and the establishment of a suitable measurement programme should be considered. In view of the uncertainty regarding the health impacts of ultrafine particles, and the recent WHO recommendations, expansion of the currently very limited monitoring of UFPs in the UK is strongly advised.
87. Future changes in emissions should also be reflected in decisions to alter or upgrade monitoring networks and in the health research conducted. For example, in regards to road traffic, the adoption of electric vehicles will have an impact on the constituents and size distribution of particles that are associated with vehicles. Insight into the potential consequences of these changes on health will be gained as progress is made in our understanding of the exposure to non-exhaust traffic-derived particles (for example, PM from brake, tyre and road wear) and their relative toxicity. The transition to Net Zero will also lead to further changes in the composition of airborne particles. There remains some uncertainty as to the precise directions of change, but declines in sulphate and nitrate particles seem likely, with a possible increase in biogenic secondary organic aerosol. Changes in particle composition may present an opportunity to evaluate effects of differing components upon health.
88. Studies in which personal monitoring of exposures includes specific particle components should be carefully considered. These have great potential but are typically limited by small sample size and hence low statistical power. There is a need to establish a greater consensus regarding the appropriate practice for research with personal monitors, in terms of monitor type, monitoring protocol, alignment with health parameters measured and data analysis methods.
Particle toxicology
89. Comprehensive studies which combine laboratory-derived measurements of particle toxicology (human controlled exposure, animal and cellular models) with population-based epidemiology, and with panel studies of human symptoms and biomarkers of exposure and effect are recommended.
90. More experimental studies are required that directly compare PM of different composition or different sources within the same study (accompanied by good characterisation of the composition). This approach would allow a comparison of components and/or sources of particulate pollution. A variety of study types should be used for this aim, for instance acellular assays (particle characterisation and potentially predictive physicochemical properties such as PM OP), in vitro studies using relevant models and exposure approaches (for instance, adverse outcome pathway assessments), in vivo exposures in animals (healthy and disease models) and controlled exposure in volunteers. The study by Gerlofs-Nijland and others (2019) is a good example of a recent mouse inhalation study directly comparing the effects of different sources of PM. Where possible, larger research programmes should utilise several of these study types to exploit the advantages of different models, for instance, advanced in vitro assays for testing greater numbers of PM types than animal or human studies can, in vivo studies to consider interactions with multiple organ systems and across generations. Omics analyses should be increasingly used to uncover new endpoints and mechanisms of toxicity following exposure to PM from different sources.
91. It is recognised that particle toxicity is mediated by a number of mechanistic pathways, and further elucidation of those pathways at a fundamental level is a high priority as it is likely to provide insights into the physical and chemical properties most important in inducing toxic reactions in the body. Toxicological studies would benefit from collection and characterisation of the ‘real-world’ samples from different measurement sites. Collection of sufficient quantities of PM would permit coordinated toxicological studies between researchers with different expertise to provide a holistic assessment of adverse effects of different constituents. This approach could also facilitate the harmonisation of methods across research groups.
92. The last 2 decades have seen the development of several methods to quantify particle-bound ROS and particle OP (Campbell and others, 2021). However, the accurate quantification of PM OP has been hindered by the lack of suitable measurement methods, as many chemical components present in PM contributing to OP are highly reactive, short-lived and in low concentrations, posing a significant analytical-chemical challenge. As a consequence, there is currently no overall agreement on the best methodology to employ to determine particulate OP (Øvrevik, 2019). Recently, online OP methods have been championed as they provide rapid, time-resolved continuous time series data in situ, thus representing a substantial improvement on traditional offline measurement methods (Campbell and others, 2019). Highly time-resolved measurements in the field will be helpful in elucidating the chemical and atmospheric drivers of OP, the spatial and temporal dynamics of OP influenced by rapidly changing atmospheric conditions and contributing sources, and thus should be helpful in drawing robust and accurate correlations between PM OP and health outcomes.
Miscellaneous
93. Differences in the susceptibility of individuals (for example, in terms of age and comorbidities) to different sources and constituents of air pollution was not addressed to any extent in the literature reviewed. This is a priority area for the air pollution mixture as a whole, and the additional focus on specific sources and constituents may yield valuable data with high relevance for public health and policy. For example, the use of OP metrics may have more relevance to vulnerable groups, such as the young and old, where adaptive mechanisms are developing or waning respectively, or within certain disease groups where there are established deficiencies in adaptations and repair mechanisms.
94. Why compositionally contrasting PM elicits seemingly similar effects on health-related outcomes is an increasingly important research question. Given the failure of research to date to resolve the key question of differential toxicity of particles, new innovative, imaginative and adventurous approaches to research are strongly encouraged.
Other considerations
95. During the final stages of drafting this statement the World Health Organization published new Global Air Quality Guidelines (AQG) (WHO, 2021), including new recommendations for guidelines for PM10 and PM2.5. Of interest, the WHO report states that “As yet, insufficient data are available to provide recommendations for AQG levels and interim targets for specific types of PM, notably BC/EC, UFP and SDS [sand and dust storms]”. Nonetheless, the 2021 WHO document does provide good practice statements for EC/BC, UFPs and SDS. We note that the ANSES report also suggested greater priority (as part of policies to monitor and reduce air pollutant emissions) should be given to BC/EC, UFPs and combustion-derived emissions. In contrast, the USEPA integrated science assessment, while noting these constituents or sources had adverse effects, did not make policy recommendations for specific constituents or sources. While the WHO good practice statements were not underpinned by a systematic review of the evidence in the same way that the AQGs were, the WHO report encourages the systematic measurement of BC/EC, as well as emissions inventories, exposure assessment and source apportionment for BC/EC. It notes, however, that these measurements should not replace or reduce existing monitoring of pollutants. The report also makes suggestions for the assessment of UFPs in terms of size ranges and particle number concentration, and promotes the use of emerging science and technology to advance approaches to the assessment of exposure to UFP for their application in epidemiological studies. These views align with the Research Recommendations we have made in this statement.
96. Finally, we remain mindful that PM2.5 is not the only air pollutant associated with adverse health effects, and that strategies to reduce primary emissions of PM will also impact levels of gaseous and secondary PM. Interventions that reduce multiple pollutants are likely to be advantageous in lessening the health burden, although the direct and indirect consequences of these interventions on the air pollution mixture and population exposure will need to be fully assessed. Nonetheless, monitoring of PM2.5 mass concentration remains an integral means to measure and regulate PM in our environment to aid efforts to reduce the health burden caused by air pollution.
COMEAP
July 2022
References
Achilleos S, Kioumourtzoglou MA, Wu CD, Schwartz JD, Koutrakis P, Papatheodorou SI. ‘Acute effects of fine particulate matter constituents on mortality: A systematic review and meta-regression analysis.’ Environment International 2017: volume 109: pages 89 to 100
Aghababaeian H, Ostadtaghizadeh A, Ardalan A, Asgary A, Akbary M, Yekaninejad MS and others. ‘Global health impacts of dust storms: a systematic review.’ Environmental Health Insights 2021: volume 15, page 11786302211018390
ANSES (2019). ‘Particulate matter in ambient air: health effects according to components, sources and particle size: impact on air pollution of the technologies and composition of the motor vehicle fleet operating in France.’ ANSES Opinion Amended summary report and recommendations from the collective expert appraisal. August 2019 Scientific Edition
Atkinson RW, Mills IC, Walton HA, Anderson HR. ‘Fine particle components and health: a systematic review and meta-analysis of epidemiological time series studies of daily mortality and hospital admissions.’ Journal of Exposure Science and Environmental Epidemiology 2015: volume 25, issue 2, pages 208 to 214
Bai L, Weichenthal S, Kwong JC, Burnett RT, Hatzopoulou M, Jerrett M and others. ‘Associations of long-term exposure to ultrafine particles and nitrogen dioxide with increased incidence of congestive heart failure and acute myocardial infarction.’ American Journal of Epidemiology 2019: volume 188, issue 1, pages 151 to 159
Campbell SJ, Utinger B, Lienhard DM, Paulson SE, Shen J, Griffiths PT and others. ‘Development of a physiologically relevant online chemical assay to quantify aerosol oxidative potential.’ Analytical Chemistry 2019: volume 91, issue 20, pages 13,088 to 13,095
Campbell SJ, Wolfer K, Utinger B, Westwood J, Zhang ZH, Bukowiecki N and others. ‘Atmospheric conditions and composition that influence PM2.5 oxidative potential in Beijing, China.’ Atmospheric Chemistry and Physics 2021: volume 21, issue 7, pages 5,549 to 5,573
Cassee FR, Morawska L, Peters A, Wierzbicka A, Buonanno G, Cyrys J and others, editors. ‘White Paper: Ambient ultrafine particles: evidence for policy makers 2019’
COMEAP (2009). ‘Long-term exposure to air pollution: effect on mortality.’
COMEAP (2015). ‘Statement on the evidence for differential health effects of particulate matter according to source or components.’
COMEAP (2020). ‘Statement on the evidence for health effects associated with exposure to non-exhaust particulate matter from road transport.’
COMEAP (2021). ‘Advice on health evidence relevant to setting PM2.5 targets.’
Downward GS, van Nunen EJHM, Kerckhoffs J, Vineis P, Brunekreef B, Boer JMA and others. ‘Long-term exposure to ultrafine particles and incidence of cardiovascular and cerebrovascular disease in a prospective study of a Dutch cohort.’ Environmental Health Perspectives 2018: volume 126, issue 12, page 127,007-
Fussell JC, Kelly FJ. ‘Mechanisms underlying the health effects of desert sand dust.’ Environment International 2021: volume 157, December 2021, page 106,790
Gerlofs-Nijland ME, Bokkers BGH, Sachse H, Reijnders JJE, Gustafsson M, Boere AJF and others. ‘Inhalation toxicity profiles of particulate matter: a comparison between brake wear with other sources of emission.’ Inhalation Toxicology 2019: volume 31, issue 3, pages 89 to 98
Godri KJ, Harrison RM, Evans T, Baker T, Dunster C, Mudway IS and others. ‘Increased oxidative burden associated with traffic component of ambient particulate matter at roadside and urban background schools sites in London.’ PLoS One 2011: volume 6, issue 7, page :e21961
HEI NPACT Review Panel (2013) ‘Executive Summary. HEI’s National Particle Component Toxicity (NPACT) Initiative.’ Health Effects Institute, Boston, MA
Hill AB. ‘The environment and disease: association or causation?’ Proceedings of the Royal Society of Medicine 1965: volume 58, issue 5, pages 295 to 300
Kelly FJ, Fussell JC. ‘Linking ambient particulate matter pollution effects with oxidative biology and immune responses.’ Annals of the New York Academy of Sciences 2015: volume 1340, pages 84 to 94
Kelly FJ, Fussell JC. ‘Toxicity of airborne particles-established evidence, knowledge gaps and emerging areas of importance. Philosophical Transactions of the Royal Society A: Mathematical, Physical and Engineering Sciences 2020: volume 378, issue 2,183, page 20,190,322
Mills NL, Miller MR, Lucking AJ, Beveridge J, Flint L, Boere AJ and others. ‘Combustion-derived nanoparticulate induces the adverse vascular effects of diesel exhaust inhalation.’ European Heart Journal 2011: volume 32, issue 21, pages 2,660 to 2,671
Mills NL, Robinson SD, Fokkens PHB, Leseman DLAC, Miller MR, Anderson D, and others. ‘Exposure to concentrated ambient particles does not affect vascular function in patients with coronary heart disease.’ Environmental Health Perspectives 2008: volume 116, issue 6, pages 709 to 715
Ohlwein S, Kappeler R, Kutlar Joss M, Kunzli N, Hoffmann B. ‘Health effects of ultrafine particles: a systematic literature review update of epidemiological evidence.’ International Journal of Public Health 2019: volume 64, issue 4, pages 547 to 559
Ostro B, Tobias A, Querol X, Alastuey A, Amato F, Pey J and others. ‘The effects of particulate matter sources on daily mortality: a case-crossover study of Barcelona, Spain.’ Environmental Health Perspective 2011: volume 119, issue 12, pages 1,781 to 1,787
Øvrevik J. ‘Oxidative potential versus biological effects: a review on the relevance of cell-free or abiotic assays as predictors of toxicity from airborne particulate matter.’ International Journal of Molecular Aci 2019: volume 20, issue 19, pages 4,772
Park M, Joo HS, Lee K, Jang M, Kim SD, Kim I and others. ‘Differential toxicities of fine particulate matters from various sources.’ Scientific Reports 2018: volume 8, issue 1, page 17,007
Rohr A, McDonald J. ‘Health effects of carbon-containing particulate matter: focus on sources and recent research program results.’ Critical Reviews of Toxicology 2016: volume 46, issue 2, pages 97 to 137
Schlesinger RB, Cassee F. ‘Atmospheric secondary inorganic particulate matter: the toxicological perspective as a basis for health effects risk assessment.’ Inhalation Toxicology 2003: volume 15, issue 3, pages 197 to 235
Selley L, Schuster L, Marbach H, Forsthuber T, Forbes B, Gant TW and others. ‘Brake dust exposure exacerbates inflammation and transiently compromises phagocytosis in macrophages.’ Metallomics 2020: volume 12, issue 3, pages 371 to 386
USEPA (2019). Integrated Science Assessment (ISA) for Particulate Matter EPA/600/R-19/188. United States Environmental Protection Agency
Weisenberg H, Zhao T, Heinrich J. ‘Combinations of epidemiological and experimental studies in air pollution research: a narrative review. International Journal of Environmental Research and Public Health 2020: volume 17, issue 2
WHO (2012). ‘Health effects of black carbon.’ WHO Regional Office for Europe, Copenhagen, Denmark
WHO (2013). ‘Review of evidence on health aspects of air pollution – REVIHAAP project: technical report.’ WHO Regional Office for Europe, Copenhagen, Denmark
WHO (2021). ‘Global air quality guidelines: particulate matter (PM2.5 and PM10), ozone, nitrogen dioxide, sulfur dioxide and carbon monoxide.’
Yang Y, Ruan Z, Wang X, Yang Y, Mason TG, Lin H and others. ‘Short-term and long-term exposures to fine particulate matter constituents and health: a systematic review and meta-analysis.’ Environmental Pollution 2019: volume 247, pages 874 to 882
Annex A: Oxidative potential of particulate matter: PubMed selected papers
-
Abrams JY, Weber RJ, Klein M, Sarnat SE, Chang HH, Strickland MJ and others. ‘Associations between ambient fine particulate oxidative potential and cardiorespiratory emergency department visits.’ Environmental Health Perspectives 2017: volume 125, issue 10, page 107,008
-
Atkinson RW, Samoli E, Analitis A, Fuller GW, Green DC, Anderson HR and others. ‘Short-term associations between particle oxidative potential and daily mortality and hospital admissions in London.’ International Journal of Hygiene and Environmental Health 2016: volume 219, issue 6, pages 566 to 572
-
Colicino E, Power MC, Cox DG, Weisskopf MG, Hou L, Alexeeff SE and others. ‘Mitochondrial haplogroups modify the effect of black carbon on age-related cognitive impairment.’ Environmental Health 2014: volume 13, issue 1, page 42
-
Delfino RJ, Staimer N, Tjoa T, Gillen DL, Schauer JJ, Shafer MM. ‘Airway inflammation and oxidative potential of air pollutant particles in a pediatric asthma panel.’ Journal of Exposure Science and Environmental Epidemiology 2013: volume 23, issue 5, pages 466 to 473
-
Janssen NA, Strak M, Yang A, Hellack B, Kelly FJ, Kuhlbusch TA and others. ‘Associations between 3 specific a-cellular measures of the oxidative potential of particulate matter and markers of acute airway and nasal inflammation in healthy volunteers.’ Occupational and Environmental Medicine 2015: volume 72, issue 1, pages 49 to 56
-
Lavigne É, Burnett RT, Stieb DM, Evans GJ, Godri Pollitt KJ, Chen H and others. ‘Fine particulate air pollution and adverse birth outcomes: effect modification by regional nonvolatile oxidative potential.’ Environmental Health Perspectives 2018: volume 126, issue 7, page 077012
-
Liu L, Urch B, Szyszkowicz M, Evans G, Speck M, Van Huang A and others. ‘Metals and oxidative potential in urban particulate matter influence systemic inflammatory and neural biomarkers: a controlled exposure study.’ Environment International 2018: volume 121 part 2, pages 1,331 1,340
-
Steenhof M, Janssen NA, Strak M, Hoek G, Gosens I, Mudway IS and others. ‘Air pollution exposure affects circulating white blood cell counts in healthy subjects: the role of particle composition, oxidative potential and gaseous pollutants: the RAPTES project.’ Inhalation Toxicology 2014: volume 26, issue 3, pages 141 to 165
-
Steenhof M, Mudway IS, Gosens I, Hoek G, Godri KJ, Kelly FJ and others. ‘Acute nasal pro-inflammatory response to air pollution depends on characteristics other than particle mass concentration or oxidative potential: the RAPTES project.’ Occupational and Environmental Medicine 2013: volume 70, issue 5, pages 341 to 348
-
Strak M, Hoek G, Godri KJ, Gosens I, Mudway IS, van Oerle R and others. ‘Composition of PM affects acute vascular inflammatory and coagulative markers: the RAPTES project.’ PloS One 2013: volume 8, issue 3, page e58944
-
Strak M, Hoek G, Steenhof M, Kilinc E, Godri KJ, Gosens I and others. ‘Components of ambient air pollution affect thrombin generation in healthy humans: the RAPTES project.’ Occupational and Environmental Medicine 2013: volume 70, issue 5, pages 332 to 340
-
Strak M, Janssen N, Beelen R, Schmitz O, Vaartjes I, Karssenberg D and others. ‘Long-term exposure to particulate matter, NO(2) and the oxidative potential of particulates and diabetes prevalence in a large national health survey.’ Environment International 2017: volume 108, pages 228 to 236
-
Strak M, Janssen NA, Godri KJ, Gosens I, Mudway IS, Cassee FR and others. ‘Respiratory health effects of airborne particulate matter: the role of particle size, composition, and oxidative potential-the RAPTES project.’ Environmental Health Perspectives 2012: volume 120, issue 8, pages 1,183 to 1,189
-
Tonne C, Yanosky JD, Beevers S, Wilkinson P, Kelly FJ. ‘PM mass concentration and PM oxidative potential in relation to carotid intima-media thickness.’ Epidemiology (Cambridge, Mass) 2012: volume 23, issue 3, pages 486 to 494
-
Weichenthal S, Crouse DL, Pinault L, Godri-Pollitt K, Lavigne E, Evans G and others. ‘Oxidative burden of fine particulate air pollution and risk of cause-specific mortality in the Canadian Census Health and Environment Cohort (CanCHEC).’ Environmental Research 2016: volume 146, pages 92 to 99
-
Weichenthal S, Lavigne E, Evans G, Pollitt K, Burnett RT. ‘Ambient PM2.5 and risk of emergency room visits for myocardial infarction: impact of regional PM2.5 oxidative potential: a case-crossover study.’ Environmental Health : a global access science source 2016: volume 15, page 46
-
Weichenthal SA, Lavigne E, Evans GJ, Godri Pollitt KJ, Burnett RT. ‘Fine particulate matter and emergency room visits for respiratory illness: effect modification by oxidative potential.’ American Journal Of Respiratory And Critical Care Medicine 2016: volume 194, issue 5, pages 577 to 586
-
Wittkopp S, Staimer N, Tjoa T, Gillen D, Daher N, Shafer M and others. ‘Mitochondrial genetic background modifies the relationship between traffic-related air pollution exposure and systemic biomarkers of inflammation.’ PloS One 2013: volume 8, issue 5, page e64444
-
Wittkopp S, Staimer N, Tjoa T, Stinchcombe T, Daher N, Schauer JJ and others. ‘Nrf2-related gene expression and exposure to traffic-related air pollution in elderly subjects with cardiovascular disease: an exploratory panel study.’ Journal Of Exposure Science and Environmental Epidemiology 2016: volume 26, issue 2, pages 141 to 149
-
Yang A, Janssen NA, Brunekreef B, Cassee FR, Hoek G, Gehring U. ‘Children’s respiratory health and oxidative potential of PM2.5: the PIAMA birth cohort study.’ Occupational and Environmental Medicine 2016: volume 73, issue 3, pages 154 to 160
-
Zhang X, Staimer N, Gillen DL, Tjoa T, Schauer JJ, Shafer MM and others. ‘Associations of oxidative stress and inflammatory biomarkers with chemically-characterized air pollutant exposures in an elderly cohort.’ Environmental Research 2016: volume 150, pages 306 to 319
-
Zhang X, Staimer N, Tjoa T, Gillen DL, Schauer JJ, Shafer MM and others. ‘Associations between microvascular function and short-term exposure to traffic-related air pollution and particulate matter oxidative potential.’ Environmental Health 2016: volume 15, issue 1, page 81
COMEAP sub-group on the differential toxicity of particulate matter according to source or constituents
Chair Dr Mark Miller
Members Professor Martin Clift
Professor Roy Harrison
Professor Frank Kelly
Secretariat Dr Artemis Doutsi
Ms Alison Gowers
Dr Naomi Earl
Dr Christina Mitsakou
COMEAP Chair (Outgoing): Professor Frank Kelly
Acknowledgements
The sub-group would like to thank Dr Matthew Loxham, University of Southampton and Dr Ian Mudway, Imperial College London for their detailed critical appraisal of an advanced draft of this statement.