NERVTAG and EMG: Role of aerosol transmission in COVID-19 - 22 July 2020
Updated 26 January 2022
Executive summary
The possibility of aerosol transmission of SARS-CoV-2 (outside of aerosol generating procedures in healthcare) has recently been formally acknowledged by WHO and hence interest in airborne transmission has increased.
EMG and NERVTAG have previously recognised the possibility of aerosol transmission of SARS-CoV-2. This paper reviews current knowledge on aerosol transmission mechanisms and mitigations to ensure that recommendations are still appropriate.
Aerosol transmission can occur when small respiratory aerosols (less than 10 micrometre diameter) containing the virus remain in the air and can be inhaled by another person. This is most likely to happen at close range (within 2 metres) though there is a small amount of evidence that this could happen in an indoor environment more than 2 metres from an infected person. There is currently no evidence for long range aerosol transmission where the virus is dispersed between rooms in a building or long distances outdoors.
It is possible that aerosol transmission plays a role in super spreading events. These are characterised by high secondary attack rates and tend to occur in poorly ventilated indoor spaces.
Good ventilation of indoor spaces will dilute and remove virus in the air. People should not spend long periods of time in poorly ventilated spaces with other people. It is recommended that organisations should take steps to ensure appropriate ventilation provision and improve ventilation or limit the occupancy of spaces that have inadequate ventilation.
Cloth face coverings are likely to have some benefit in reducing the risk of aerosol transmission. Face coverings will reduce the dispersion of respiratory droplets and small aerosols that carry the virus into the air from an infected person. They also provide a small amount of protection for the wearer against exposure to droplets but less protection against small aerosols.
Asymptomatic and presymptomatic transmission of SARS-CoV-2 is now known to occur. Thus people without symptoms who are potentially infectious to others may be in public places. For this reason, it is advisable to encourage face covering in addition to ventilation, social distancing and hand hygiene to interrupt transmission. They should particularly be encouraged in indoor environments with poor ventilation or when large numbers of people congregate to reduce the risk of super spreading events.
Fit-tested FFP3 respirators provide a higher level of protection to the wearer against aerosol transmission. However the evidence that aerosol transmission is significant compared to other routes is not sufficiently strong to recommend that these are used in locations other than high risk clinical areas where aerosol generating procedures take place.
Face shields or visors are unlikely to be an effective control for aerosol transmission. Face shields provide protection for the wearer against large droplet exposure, including by inoculation through the eyes. However, they are unlikely to provide any protection for the wearer against small aerosols. There is no evidence that face shields or visors are an effective source control for either larger droplets or small aerosols.
It is recommended that guidance for settings where people are in close proximity for a long duration (for example hairdressing), and that currently only require face shields to be worn, should be changed to include the wearing of face coverings.
Although the focus of this paper is on aerosol transmission, transmission through droplets at close-range and contact with surfaces remain important mechanisms. Other measures including physical distancing, good hand hygiene and cleaning of surfaces therefore remain very important infection control measures. The relative importance of these three routes of transmission is not yet clear.
Rationale for reviewing aerosol transmission
The Environment and Modelling subgroup of SAGE identified the potential for aerosol transmission of SARS-CoV-2 in its first paper titled ‘Evidence of environmental dispersion for COVID-19 for different mechanisms (14 April 2020)’. The possibility that SARS-CoV-2 could be airborne was recognised by NERVTAG in its minutes of 13 January 2020. On 9 July 2020 WHO issued updated guidance on the transmission of the SARS-CoV-2 and implications for infection prevention[footnote 1]. A letter signed by 239 scientists and published in Clinical Infectious Diseases [footnote 2]calls for aerosol transmission to be recognised and is likely to have prompted WHO to change its guidance.
The updated WHO guidance acknowledges that in poorly ventilated spaces, transmission through an airborne route cannot be ruled out. This is a notable change from previous guidance which indicated the virus was spread by droplet and contact transmission, with airborne transmission only a concern in hospital settings where aerosol generating procedures were carried out. The same updated guidance also confirms that transmission can happen before people develop symptoms or when they remain asymptomatic.
Terminology surrounding droplets and aerosols can be confusing, with different definitions used within different research communities. Within this paper we use the following definitions:
-
Aerosol – a small respiratory particle that carries the virus and can remain in the air for a long period of time (at least 5 minutes, in many cases hours). These typically have a diameter less than 10 micrometres.
-
Droplet – a larger respiratory particle that carries the virus and will normally settle out of the air in less than 5 minutes. These typically deposit on people and surfaces less than 2 metres from the source. The majority of respiratory droplets are between 10 and 100 micrometres in diameter, but a small number can be up to 1500 micrometres.
The highest risk for transmission is when people are in close proximity (less than 2 metres). This close-range transmission may be due to a combination of droplets and aerosols, as well as contaminated surfaces, and it is not yet possible to determine which mechanisms are dominating this transmission. This means that physical distancing, good hand hygiene and cleaning of surfaces remain very important infection control measures.
Short-range aerosol transmission can occur when small aerosols carrying the virus are exhaled by an infected person and remain suspended in the air for a period of time without significant dilution. If a sufficient number are inhaled this could cause infection in a susceptible individual. These aerosols can remain in the air for prolonged periods due to air currents in a room. Aerosol infection risk is highest for those in close proximity to the infected person as the aerosols are more concentrated close to the source. However, because the air disperses the particles, there is a risk to people who are beyond 2 metre distance. Risk of infection will be time dependant.
Based on the current evidence, it is possible that transmission through aerosols could happen where a person who generates significant amounts of virus is in a poorly ventilated space with others for a significant amount of time. There is no evidence of long-range airborne transmission (for example between different rooms in a building), such as occurs with measles and Tuberculosis (TB). SARS-CoV-2 is thought to be opportunistically airborne, with a risk of transmission through the air most likely to be within the same room and possibly the immediate neighbouring spaces.
Evidence for potential for aerosol transmission
Studies on respiratory exhalations:
Several studies have measured the generation of respiratory droplets and aerosols and shown that particles from less than 1 micrometre to over 1000 micrometre in diameter can be generated through respiratory activities, with a high proportion of particles in the 1 micrometre to 20 micrometre size range. The amount generated depends on the activity; coughing and sneezing are higher than breathing and talking and there is evidence that louder speech increases the amount of particles exhaled [footnote 3]. Ongoing studies in the USA, within PHE and through DCMS confirm the increase in aerosols with the loudness of singing and speech, and also show significant variation between people. This is also suggested by analysis of influenza outbreaks which suggest more than three orders of magnitude variation in respiratory output between individuals [footnote 4]. PHE data shows that in a poorly ventilated small room, aerosols carrying oral bacteria are dispersed evenly throughout the air in the room.
Laboratory aerosol studies:
Laboratory studies have reported that under experimental conditions (aerosolisation into a closed chamber) SARS-CoV-2 is stable in air for over 3 hours with very little loss of viability[footnote 5]. A recent study reports that SARS-CoV-2 survival in air is greater than SARS and MERS, and suggests infectivity could be retained for up to 16 hours in respirable sized aerosols[footnote 6]. However, survival of viable virus in air is affected by the conditions, such as humidity, temperature and UV light exposure. A study with the virus suspended in aerosolised simulated saliva reports that humidity has a minor effect on the decay rate, but that UV in sunlight is important. The time for 90% decay under US summer and spring or autumn conditions were estimated at 8 and 19 minutes respectively, compared with 286 minutes in the absence of UV light. The authors suggest aerosol transmission risk could depend on environmental conditions[footnote 7].
Animal studies:
Experiments conducted with ferrets and hamsters demonstrate that transmission is possible between animal cages with no direct interactions[footnote 8] [footnote 9][footnote 10]. However, it is not possible to determine whether this transmission is through small aerosols or larger droplets that are capable of dispersing some distance, since the distances between animals are limited to centimetres.
Data from clinical settings:
Several studies have attempted to sample the virus from the air in clinical environments. The majority of air samples have been negative, but in some cases viral RNA copies have been measured in patient rooms, patient toilets, staff areas and areas where PPE is removed. The maximum levels of airborne RNA detected has been 7,000 copies per cubic metre with levels reported as low as 1 copy number per cubic metre. One study showed detection of viral RNA in the air in crowded public areas, including an entrance to a hospital building [footnote 11]. A new pre-print claims to have measured infectious virus from the air in a hospital, but this work has yet to be peer reviewed and a number of virology experts have expressed doubt over the work [footnote 12]. In many these studies the air samplers used are unable to discriminate between aerosol and droplet particles.
Evidence from outbreaks:
There are a number of outbreaks where there is a very high secondary attack rate over a short period of time. These include documented information on a choir[footnote 13], fitness class [footnote 14] and restaurant outbreak [footnote 15] as well as more recent anecdotal data from a church where participants reportedly complied with social distancing and hand hygiene [footnote 16]. While droplet and surface contact transmission can’t be excluded for these cases, it is difficult to fully explain the level of transmission without considering that airborne routes are playing a part.
Modelling studies:
A pre-print modelling the Skagit choir outbreak as an aerosol transmission model suggests very high viral emission rates (higher than normally calculated for influenza and TB) may have played a role[footnote 17]. A recent hospital scenario modelling study attempts to evaluate the relative modes of transmission, and suggests that inhalation is significant mechanism, but doesn’t clearly differentiate between droplets and aerosols[footnote 18]. While there is no conclusive evidence for airborne transmission of SARS-CoV-2, it should be noted that there is no robust proof for close-range droplet or surface contact transmission either. However, there is substantial data indicating that SARs-CoV-2 transmission risk is highest with close contact in enclosed settings, with households posing a particular risk.
Control measures for airborne infection
SAGE EMG has already considered that the virus could be transmitted through airborne routes and has included this in relevant papers on transmission and recommendations for mitigating risk. NERVTAG have previously considered asymptomatic transmission. However the statements by WHO have raised public awareness of the potential risks associated with airborne transmission and hence the implications for infection control are briefly summarised here. Further evidence and information on these measures are in previous EMG and NERVTAG papers.
Ventilation and air conditioning
Good ventilation is well recognised as a primary measure for controlling the risk of airborne disease transmission. A well ventilated space reduces the concentration of viral load in the air and hence the probability of infection, as illustrated in figure 1. Evidence to date suggests that poorly ventilated spaces pose the highest risk, so it is recommended that mitigation measures focus on those spaces where ventilation is absent or inadequate. Those spaces where there are several people in close proximity for a period of 30 minutes or more (for example social spaces, schools and university lecture rooms, meeting rooms, performance spaces) or where an infectious person is more likely to be present (such as GP surgeries, pharmacies) should be prioritised for mitigation.
Ventilation refers to provision of sufficient fresh (outdoor) air, while air conditioning is provided to control the temperature in a space. Building regulations and Health and Safety at Work legislation require adequate ventilation to be provided in all domestic, public and commercial buildings. The building regulations and guidance provided by CIBSE recommends 10 litre per second per person fresh air is provided in most public and commercial buildings. Requirements for domestic ventilation set out in the building regulations depend on the size of the property and provide whole house rates, but at best are 7 litre per second per person. These standards only apply to recent buildings and there are large numbers of spaces across the breadth of UK building stock where the provision of ventilation does not comply with the building regulations.
It is recommended that ventilation in all occupied public spaces should at least meet the current building regulations for the space. Those spaces which are occupied by more than one person and do not meet this standard should be have their ventilation upgraded where possible, or the use of the space restricted to a lower occupancy to reduce overall risk of transmission. Mechanical ventilation systems should be operated at the maximum design flow rate, even if a space has a lower occupancy than the maximum permitted. As set out in CIBSE guidance [footnote 19] it is recommended that ventilation systems are set to run on full fresh air as far as possible.
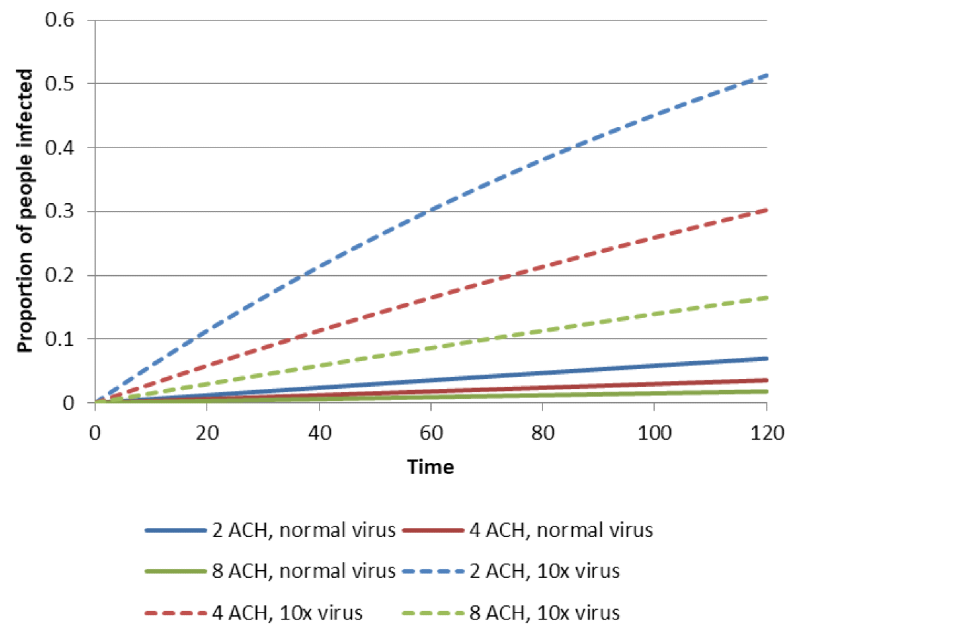
Figure 1: Typical relationship between infection risk, duration of exposure, ventilation rate and viral shedding rate based on the Wells-Riley model for airborne transmission. Values are based on a ventilation rate of 2 to 8 ACH in a 500 cubic metre room with an occupant breathing rate of 10 litres per minute. A ‘normal’ viral shedder has a quanta generation rate of 1 quanta per hour as calculated by [footnote 20].
Several commentators have suggested that recirculating air conditioning units pose a risk for transmission. It is possible that these units (and other flow devices such as fans) could maintain droplets in the air for longer and propel them greater distances. However, the underlying issue in many spaces is most likely to be one of poor fresh air ventilation. Air conditioning units can mask issues of poor ventilation by cooling a space that would otherwise overheat due to the lack of fresh air and may lead to the closure of windows. As such, it is likely that using air conditioning units in well-ventilated spaces does not significantly influence the risk of transmission. Further research to confirm the influence of air conditioning units on different sizes of respiratory particles would be valuable.
The use of recirculating air cleaners may be appropriate in small spaces where ventilation is poor and cannot be easily improved. Devices which use HEPA or UV-C are likely to be the most effective. There is limited high quality evidence for other technologies and some can pose a hazard (for example by introducing ozone). It should be noted that these devices will only deal with the contaminant and will not address the underlying issues of inadequate ventilation. These have been considered in the EMG paper ‘Summary of disinfection technologies for microbial control and repository paper 18 May 2020’.
Ensuring good ventilation of buildings is a particular concern for winter [footnote 21], where cold or adverse weather means that ventilation rates are often reduced to manage thermal comfort. This is a particular problem in naturally ventilated buildings which rely on user behaviour to open windows and vents, which can present problems of thermal comfort, noise and security. It is recommended that owners and occupiers put plans in place for ensuring good ventilation is maintained whilst recognising these issues. This could include providing information to occupants on using ventilation systems effectively and providing additional heating in some locations to counter additional heat losses.
Face-coverings and masks
As source control: Most types of cloth face coverings and masks are likely to provide some benefit as a source control against both larger droplets and small aerosols. Small aerosols (less than 10 micrometres) that carry virus and can remain airborne in the air in a room may start life as much larger respiratory droplets (more than 10 micrometres). These evaporate when they leave the mouth and are exhaled into the less humid air in an indoor environment. Studies suggest that the final size of an aerosol which has evaporated is around 20 to 40% of its starting diameter depending on the humidity[footnote 22]. Hence, a 30 micrometre droplet in a dry environment could evaporate to become a 6 micrometre aerosol. Although a cloth face covering or surgical mask is not likely to be effective at trapping small aerosols, they are likely stop these larger droplets that would go on to become the small aerosols as there is no time for them to evaporate. As such, they are likely to reduce the source of both larger droplets and small aerosols. Face coverings and masks will be less effective at preventing the release of smaller aerosol particles that are less than 5 micrometres when exhaled. A study of the influence of surgical facemasks on exhalation from people with influenza shows a 25-fold reduction in aerosols less than 5 micrometres and a 2.8-fold reduction for aerosols more than 5 micrometres[footnote 23]. An unpublished PHE study considering the reduction effect of masks against exhalation of oral bacteria when coughing shows cloth face masks to be as effective as surgical masks. A report of lack of transmission from two COVID-19 infected hairdressers in the US to 139 clients in a hair dressing salon, when both the stylists and clients and wore facemasks or coverings [footnote 24] is evidence of the potential benefit of these in reducing transmission. Similarly, there is anecdotal evidence that nosocomial transmission of COVID-19 has reduced since the extended use of facemasks by healthcare workers was implemented in hospitals in England and the US [footnote 25]. However, in both of these cases it is not clear whether transmission is through droplets or aerosols.
As protection for the wearer: Face coverings are likely to provide some protection for the wearer from exposure to large droplets via the nose and mouth, but are unlikely to reliably protect against the inhalation of small aerosols although laboratory studies show they may have a small effect [footnote 26]. Well-fitting respiratory protective equipment which meets the standards for FFP3 or FFP2 are shown to be effective at stopping small aerosols. However these are only considered to be appropriate where there is a significant risk of aerosol transmission, for example during aerosol generating procedures in a healthcare or dental setting.
As current evidence suggests that the highest likelihood of aerosol transmission, outside of high risk clinical spaces, is from an asymptomatic infector in a poorly ventilated space, enhanced use of face coverings in indoor spaces is recommended. Face coverings are not usually in scope of personal protective equipment (PPE) regulations, which has meant that there has been no standard against which performance can be checked. However, recent work by CEN, the European Committee for Standardization, has produced a guide to community face coverings (CWA 17553:2020) which specifies the minimum requirements for reusable or disposable community face coverings and materials intended for the general public, including adults and children aged 3 to 12 (when being supervised by an adult) [footnote 27].
Face shields or visors
Face shields or visors are likely to be effective at protecting the wearer from exposure to large droplets, and unlike face coverings also provide protection for exposure through the eyes. However they are unlikely to provide any protection against inhalation of aerosols. There is also no evidence for them as a source control for either larger droplets or small aerosols. An anecdotal report from an outbreak in Switzerland has suggested those wearing face shields were more likely to be infected than those wearing masks, however this should be treated with caution as the outbreak investigation has not yet formally reported. Current evidence suggests that face shields are not an effective measure against airborne transmission.
Recommendations
Steps should be taken to ensure good ventilation in all buildings to mitigate aerosol transmission. Priority should be given to spaces where ventilation is absent or inadequate, those where there are several people in close proximity for a period of 30 minutes or more and those where an infectious person is more likely to be present (for example GP surgeries, pharmacies).
Particular attention should be paid to planning for winter to ensure that spaces can be effectively ventilated without significantly compromising the thermal comfort of occupants.
Greater use of cloth face coverings should be encouraged in indoor environments with poor ventilation or when large numbers of people congregate to reduce the risk of super spreading events.
Given emerging evidence on face shields and the recent study from a hairdressing salon in the US, we recommend that guidance for UK hairdressers and barbers should be strengthened to include wearing of face coverings.
There is a need for further research to understand the best strategies for controlling airborne transmission risk, particularly in public buildings where there is high occupancy over a long period of time. Research to understand the influence of air conditioning units on different particle sizes would be valuable.
There is a need for further research to understand the effectiveness of face shields or visors as a mitigation measure.
References
-
WHO ‘Transmission of SARS-CoV-2: implications for infection prevention precautions,’ 9 July 2020 ↩
-
L. Morawska et al., ‘How can airborne transmission of COVID-19 indoors be minimised?,’ Environ. Int., vol. 142, no. January, p. 105832, Sep. 2020, doi: 10.1016/j.envint.2020.105832. ↩
-
S. Asadi, A. S. Wexler, C. D. Cappa, S. Barreda, N. M. Bouvier, and W. D. Ristenpart, ‘Aerosol emission and superemission during human speech increase with voice loudness,’ Sci. Rep., vol. 9, no. 1, pp. 1–10, 2019, doi: 10.1038/s41598-019-38808-z. ↩
-
P. J. B. De Mesquita, C. J. Noakes, and D. K. Milton, ‘Quantitative aerobiologic analysis of an influenza human challenge-transmission trial,’ Indoor Air, vol. Under revi, 2020. ↩
-
N. van Doremalen et al., ‘Aerosol and Surface Stability of SARS-CoV-2 as Compared with SARS-CoV-1,’ N. Engl. J. Med., Mar. 2020, doi: 10.1056/nejmc2004973. ↩
-
A. C. Fears et al., ‘Persistence of Severe Acute Respiratory Syndrome Coronavirus 2 in Aerosol Suspensions,’ Emerg. Infect. Dis., vol. 26, no. 9, Sep. 2020, doi: 10.3201/eid2609.201806. ↩
-
M. Schuit et al., ‘Airborne SARS-CoV-2 is Rapidly Inactivated by Simulated Sunlight,’ J. Infect. Dis., 2020, doi: 10.1093/infdis/jiaa334. ↩
-
Y. Il Kim et al., ‘Infection and Rapid Transmission of SARS-CoV-2 in Ferrets,’ Cell Host Microbe, vol. 27, no. 5, pp. 704-709.e2, 2020, doi: 10.1016/j.chom.2020.03.023. ↩
-
S. F. Sia et al., ‘Pathogenesis and transmission of SARS-CoV-2 in golden hamsters,’ Nature, 2020, doi: 10.1038/s41586-020-2342-5. ↩
-
M. Richard et al., ‘the air between ferrets,’ Nat. Commun., no. 2020, pp. 1–6, 2019, doi: 10.1038/s41467-020-17367-2. ↩
-
Y. Liu et al., ‘Aerodynamic analysis of SARS-CoV-2 in two Wuhan hospitals,’ Nature, vol. 86, no. 21, p. 2020.03.08.982637, 2020, doi: 10.1038/s41586-020-2271-3. ↩
-
J. L. Santarpia et al., ‘The Infectious Nature of Patient-Generated SARS-CoV-2 Aerosol,’ no. Cdc, 2020. ↩
-
L. Hamner et al., ‘High SARS-CoV-2 Attack Rate Following Exposure at a Choir Practice — Skagit County, Washington, March 2020,’ MMWR. Morb. Mortal. Wkly. Rep., vol. 69, no. 19, pp. 606–610, 2020, doi: 10.15585/mmwr.mm6919e6. ↩
-
S. Jang, S. H. Han, and J.-Y. Rhee, ‘Cluster of Coronavirus Disease Associated with Fitness Dance Classes, South Korea,’ Emerg. Infect. Dis., vol. 26, no. 8, pp. 1917–1920, 2020, doi: 10.3201/eid2608.200633. ↩
-
J. Lu et al., ‘COVID-19 Outbreak Associated with Air Conditioning in Restaurant, Guangzhou, China, 2020,’ Emerg. Infect. Dis., vol. 26, no. 7, pp. 1628–1631, 2020, doi: 10.3201/eid2607.200764. ↩
-
S. L. Miller et al., ‘Transmission of SARS-CoV-2 by inhalation of respiratory aerosol in the Skagit Valley Chorale superspreading event,’ medRxiv, no. June, p. 2020.06.15.20132027, 2020, doi: 10.1101/2020.06.15.20132027. ↩
-
R. M. Jones, ‘Relative contributions of transmission routes for COVID-19 among healthcare personnel providing patient care.,’ J. Occup. Environ. Hyg., vol. 0, no. 0, pp. 1–8, 2020, doi: 10.1080/15459624.2020.1784427. ↩
-
G. Buonanno, L. Stabile, and L. Morawska, ‘Estimation of airborne viral emission: Quanta emission rate of SARS-CoV-2 for infection risk assessment,’ Environ. Int., vol. 141, no. April, p. 105794, 2020, doi: 10.1016/j.envint.2020.105794. ↩
-
Academy of Medical Sciences, Preparing for a Challenging Winter ↩
-
M. Nicas, W. W. Nazaroff, and A. Hubbard, ‘Toward understanding the risk of secondary airborne infection: Emission of respirable pathogens,’ J. Occup. Environ. Hyg., vol. 2, no. 3, pp. 143–154, 2005, doi: 10.1080/15459620590918466. ↩
-
D. K. Milton, M. P. Fabian, B. J. Cowling, M. L. Grantham, and J. J. McDevitt, ‘Influenza Virus Aerosols in Human Exhaled Breath: Particle Size, Culturability, and Effect of Surgical Masks,’ PLoS Pathog., vol. 9, no. 3, 2013, doi: 10.1371/journal.ppat.1003205. ↩
-
M. J. Hendrix, C. Walde, K. Findley, and R. Trotman, ‘Absence of Apparent Transmission of SARS-CoV-2 from Two Stylists After Exposure at a Hair Salon with a Universal Face Covering Policy — Springfield , Missouri , May 2020,’ vol. 101, no. May, pp. 9–11, 2020. ↩
-
Wang X, et al. Association between universal masking in a health care system and SARS-CoV-2 positivity among health care workers. JAMA, 14 July 2020. ↩
-
C. Makison Booth, M.Clayton, B. Crook, J.M. Gawn Effectiveness of surgical masks against influenza bioaerosols. J Hosp Infect 2013, 84(1): 22-26 ↩