EMG: Application of physical distancing and fabric face coverings in mitigating the B117 variant SARS-CoV-2 virus in public, workplace and community - 13 January 2021
Updated 13 May 2022
Executive summary
This paper mainly focuses on physical distancing and fabric face coverings. It is important to remember these must be applied alongside other measures to address all routes of transmission, using a hierarchy of risk controls approach to determine the most suitable mitigations for public, workplace and community settings. The paper does not cover healthcare settings.
- Physical distancing and use of fabric face coverings, alongside other interventions, are important mitigation strategies to reduce community transmission of SARS-CoV-2, and are likely to be needed to be applied more consistently and effectively to be able to mitigate transmission of the B117 variant (high confidence).
- Face coverings can be effective in reducing transmission in public and community settings (medium confidence). Their effectiveness stems mostly from reducing the emission of virus-carrying particles when worn by an infected person (source control). They may provide a small amount of protection to an uninfected wearer; however, this is not their primary intended purpose (medium confidence).
- Face coverings (worn correctly and of suitable quality) are likely to be most effective at reducing transmission in both indoor and outdoor settings when people are likely to be close together (high confidence). There may be marginal benefits in some indoor spaces where people are further apart through the reduction in the amount of small aerosols released into the space (low confidence).
- Distancing between people reduces risk of exposure to all virus containing droplets and aerosols, with a significant reduction in exposure by around 2m. Exposure beyond 2 metres is mainly determined by ventilation. Maintaining a 2 metre distance where possible is likely to be more important than previously (medium confidence).
- Additional mitigations will be required at distances below 2 metres that reduce direct exposure to respiratory particles (high confidence). This needs to be determined through an appropriate consideration of risks in the environment and could include face coverings, face visors, well designed screens or barriers placed between people, and reducing the duration of exposure.
- As a precautionary measure it is recommended that public advice on wearing of fabric face coverings should be strengthened to more effectively promote their correct wearing, good hygiene practices associated with their use, and advice on selection of effective face coverings (medium confidence).
- Consideration should be given to using face coverings in a wider range of settings where people could be asymptomatic and may be in close proximity (< 2 metres). This may include outdoor spaces where it is difficult to maintain distance and people may be close together for extended periods (medium confidence).
- Messages to support application of both distancing and use of face coverings need to consider inequalities and access to mitigations, and be developed and evaluated with input from leaders from all affected organisations and communities (high confidence).
Evidence summary
1. SARS-CoV-2 transmission occurs through exposure to virus in respiratory particles. This mainly happens at close-range, and to a lesser extent via particles in the air at longer distances in indoor environments or via contact with contaminated surfaces (medium confidence).
2. Although there are uncertainties in the reasons why the B117 virus variant is more transmissible, the behaviour of respiratory particles is not likely to have changed (high confidence). It is possible that transmission can happen with a reduced duration of exposure to someone who is infectious (medium confidence). Physical distancing and use of face coverings are therefore both important mitigation strategies to reduce transmission of SARS-CoV-2. However, these mitigation measures are likely to be needed to be applied more consistently and effectively to be able to mitigate transmission of the B117 variant (high confidence).
3. This document only covers the role of distancing, face coverings and face visors in public, community and workplace settings. It does not cover the use of face coverings or visors in healthcare environments. Face coverings may be beneficial in household settings where there is a suspected or confirmed case, however this use is not explicitly considered in this paper.
4. Face coverings are defined as cloth masks or other forms of fabric barrier designed to cover the nose and mouth. They are typically made of 1 to 3 layers of cotton or man-made fabric with ties or ear loops. Some designs also incorporate some form of additional filter material. Medical style face coverings routinely worn by members of the public may also come under this category – although many look like medical masks, only some of those sold to the public (those marked type 2 or 2R) are manufactured to the same standards as the medical masks used in healthcare settings. Face coverings also need to be distinguished from respirator masks used as personal protective equipment. Face visors (or shields) are transparent plastic devices which cover the whole face including the eyes.
5. It is important to remember that face coverings and maintaining distance must be applied alongside other measures to address all routes of transmission including: ventilation; hand washing and cleaning of surfaces; actions to reduce the duration of exposure and control the number of people within spaces; and effective testing, tracing and isolation (high confidence).
6. Face coverings worn in public, community and workplace settings and used alongside other interventions can be effective in reducing transmission (medium confidence). Their effectiveness stems mostly from reducing the emission of virus-carrying particles when worn by an infected person – known as source control. They are likely to effectively reduce the emission of droplets (> 100 micrometres) and larger aerosols (10 to 100 micrometres) from an infected individual (high confidence), and may also partially reduce the emission of smaller aerosols (< 10 micrometres) if they are of a good quality and are worn correctly (medium confidence).
7. Widespread application of face coverings as a source control is likely to have a small but significant impact on population level transmission, through reducing the shedding of virus by people who may be unknowingly infected and without symptoms. The benefit is difficult to quantify, but estimates range from 7% to 45%. It is difficult in these studies to separate out the effect of face coverings compared to other interventions used at the same time (medium confidence).
8. Face coverings may provide some protection to an uninfected wearer against respiratory particles and through reducing face touching. However, this is very dependent on their design and how well they are worn. It is not their primary intended purpose and there is insufficient evidence to assume that they can provide effective protection to wearers when used for this purpose (medium confidence).
9. Face coverings are likely to be most effective at reducing transmission in both indoor and outdoor settings where people are not able to socially distance effectively and consistently so they may be close together and hence be likely to expose other people to droplets and larger aerosols. To deliver this benefit the masks must be of a suitable quality and be worn appropriately (fitting tightly to both the nose and mouth) (high confidence). This is likely to include public spaces where the interaction of people is less controlled, and communal and circulation spaces in workplaces where other measures determined by the risk assessment and the hierarchy of control have failed to reduce the risk sufficiently. There may be some small benefits in some indoor spaces where people are further apart through the reduction in the amount of small aerosols released into the space, which will be more significant if people are correctly wearing effective face coverings (low confidence). This benefit from the reduction of small aerosols is not likely to be significant in outdoor environments (high confidence), but the reduction in larger droplets may be important at close range in outdoor environments (medium confidence).
10. Face visors are a physical barrier designed to protect the wearer and are not a direct alternative to a face covering. They are likely to be effective in partially protecting the wearer from larger aerosols and droplets emitted by an infected person but are unlikely to be effective in protecting against smaller aerosol particles (medium confidence). There is no published evidence that they are infection from a wearer, but it is possible that they may be able to capture some larger droplets and aerosol particles that are exhaled by an infected person through impaction on the inside of the visor (low confidence).
- 11. There is no specific evidence relating to the effectiveness of face coverings or face visors in the context of the B117 variant. Given the variant’s higher transmissibility, as a precautionary measure, the following are recommended:
- a. Advice on the correct wearing of face coverings should be strengthened to more effectively promote: wearing of face coverings in ways that improve their effectiveness as source controls; good hygiene practices including hand hygiene following removal of the face covering; as regular washing or disposal of face coverings.
- b. Provision of clearer advice on selection of effective face coverings would assist the public in making choices that are more likely to reduce transmission. It would be beneficial to work towards standards for face coverings to enable members of the public and employers to select more effective products; a kitemark approach already exists but there is little awareness of it (BSI 2020).
- c. It would be beneficial to clearly articulate the difference between the role of a face covering and face visor
12. Distancing between people reduces risk of exposure to all sizes of virus containing droplets and aerosols, with a significant reduction in exposure of around 2 to 10 times at 2 metres compared to 1 metre. It is important to recognise that it is not possible to guarantee a completely ‘risk-free’ distance within a shared indoor space – a greater distance for a given duration of exposure simply reduces the probability of exposure to the virus (high confidence). In an indoor space the risk beyond 2 metres is mainly through small aerosols that can remain suspended in the air and is determined by the ventilation rate and air flow patterns. Therefore, there is little benefit to distancing beyond 2 metres (high confidence).
13. There is no specific evidence relating to the effectiveness of distancing in the context of the B117 variant, however the way respiratory particles behave in the air does not change. It is possible that the risk of transmission at all distances and from all particle sizes may be higher than with previous variants. Therefore, maintaining a 2 metre distance where possible is likely to be more important than previously (medium confidence).
14. Transmission in outdoor settings where people are distanced is likely to still be very low risk. However, it remains the case that if people are in close proximity for extended periods in an outdoor setting, there is a potential risk of transmission from the higher concentrations of respiratory particles near to an infected person. It is possible that this close-range risk is greater with the B117 variant (low confidence).
- 15. Transmission risk is likely to increase substantially at distances below 2m, especially if interactions happen over longer periods of time. If it is not possible to maintain distances of 2m, then additional mitigations will be required that reduce direct exposure to respiratory particles (high confidence). In indoor spaces these may include one or both of the following:
- a. Face coverings can reduce exposure risk through reducing the source of respiratory particles from an infected person as detailed above. They are also likely to reduce the distance that exhaled breath can carry higher concentrations of particles by dispersing more evenly around a person’s head.
- b. Face visors can protect a wearer from droplets and larger aerosols exhaled by an infected person, as detailed above.
- c. Positioning people so they are not face to face may have some benefits, however this may not happen reliably in many environments as people will move.
- d. Well-designed physical screens and barriers positioned between 2 people are likely to significantly reduce exposure to droplets and larger aerosols (medium confidence). Their impact on exposure to small aerosols is less certain and is likely to depend on their design and position relative to the room ventilation (low confidence).
16. Reducing the duration of exposure is an important control measure, and will minimise the probability of exposure to all respiratory particles through all transmission routes. A very short duration exposure (for example, passing in a corridor or the street) will pose a very low risk of exposure to the virus compared to spending an extended period of time in close proximity to someone (high confidence).
17. Consideration should be given to using face coverings in a wider range of settings where people could be asymptomatic and are in close proximity (<2 metres), or where the environment or behaviours mean distancing is likely to be difficult. This includes outdoor spaces which are crowded or where it is difficult to maintain distance, especially if these are semi-enclosed (for example, outdoor seating areas and markets) (medium confidence).
18. Application of additional measures may be required in some workplaces where people are required to remain very close together for long periods of time. Measures may include workplace bubbling, measures to rapidly identify potential infections and support effective isolation and as a last resort, suitable personal protective equipment (PPE) which complies with appropriate standards for the function (for example correctly designed and worn face visors and/or medical grade masks, supported with appropriate training). This would be identified through completion of a suitable and sufficient COVID-secure risk assessment which had considered all other potential mitigations using the hierarchy of risk controls.
19. Increased cleaning and enhanced hand hygiene are important control measures to mitigate the higher surface contamination that may be present in close proximity to an infected person, but they are not sufficient on their own to mitigate the risk from close-range transmission (high confidence).Wearing of face coverings may reduce the contamination of surfaces through reducing the emission of droplets and larger aerosols which may deposit on surfaces (medium confidence).
- 20. Messages to support application of both distancing and use of face coverings are likely to be more effective when developed and evaluated with input from leaders from all affected communities, including local leaders. It is important that key messages set the use of face coverings and distancing in the context of the hierarchy of risk controls and other measures. Key messages relating to this paper are:
- a. there are no magic bullets – maintaining distance and wearing face coverings are important as part of a package of measures that reduce the risk of infection, but will not completely eliminate risk
- b. wearing a face-covering is important to protect other people because it reduces the risk of passing on the virus to others
- c. face-coverings are likely to be more effective when they are constructed from 2 to 3 layers of high-quality materials, are clean and fit tightly over nose and mouth to prevent virus escaping
- d. if someone is infected or quarantining it is particularly important that they wear a good quality face-covering if they have to spend any time in spaces used by other people
- e. touching a face-covering could contaminate hands with virus, and so hands should be cleaned regularly and particularly after removing or adjusting a face-covering before touching anything else to prevent virus spreading to other surfaces (for example doors, handrails, phones)
- f. in shared indoor spaces, there is no guaranteed safe distance, but maintaining a distance around 2 metres as far as possible is likely to reduce the risk of infection. Very short duration closer exposures are unlikely to pose a significant risk, especially outdoors
- g. if close range interaction is unavoidable, additional mitigations are needed to reduce the risk of transmission, and these must consider how the virus spreads to be designed properly.
21. It is important to continue to recognise that there are a small number of people who have health conditions meaning that they are unable to wear face coverings and advice must continue to recognise and support these people. Inequalities and access to face coverings should also be considered, including that many people who may be more at risk of COVID-19 may be those least able to afford or access effective face coverings.
22. There remain several areas where the evidence base is weak or absent including: the amount of virus exhaled by people and in different sizes of particles; the relative importance of different routes of transmission in different circumstances; the amount of virus needed to cause infection; and, the relative effectiveness of different mitigation measures in different circumstances. The advice provided in this paper is based on the evidence available at the time of writing, drawing from ecological studies, laboratory studies, modelling studies and emerging early understanding of the B117 variant.
Supporting evidence
Virus transmission and the B117 variant
23. The SARS-CoV-2 virus is contained within particles emitted through all respiratory activities of an infected person. Transmission to another person is through their exposure to these respiratory particles and can happen at close-range, via the air, or via contact with contaminated surfaces. To characterise exposure within this paper it is helpful to group these particles into four overlapping categories. This follows understanding from exposure science (Milton, 2020), and the behaviour in air of these different particle sizes. This is illustrated in Figure 1 and discussed in more detail in Annex A.
- respirable aerosols are < 5 μ m, will remain airborne for long periods and can penetrate to the deep lung on inhalation
- thoracic aerosols are 5 to 15 μ m, will often remain airborne over more than 2 metres and can penetrate the thorax on inhalation
- nasopharyngeal (upper airway) aerosols are 15 to 100 μ m, will normally only remain airborne for 1 to 2 metres unless air velocities are high, and will deposit in the nasal cavities and mouth following inhalation or can deposit on mucous membranes
- droplets are particles >100 μ m. They behave ballistically, normally depositing within 2m, and can cause infection by direct deposition onto mucous membranes
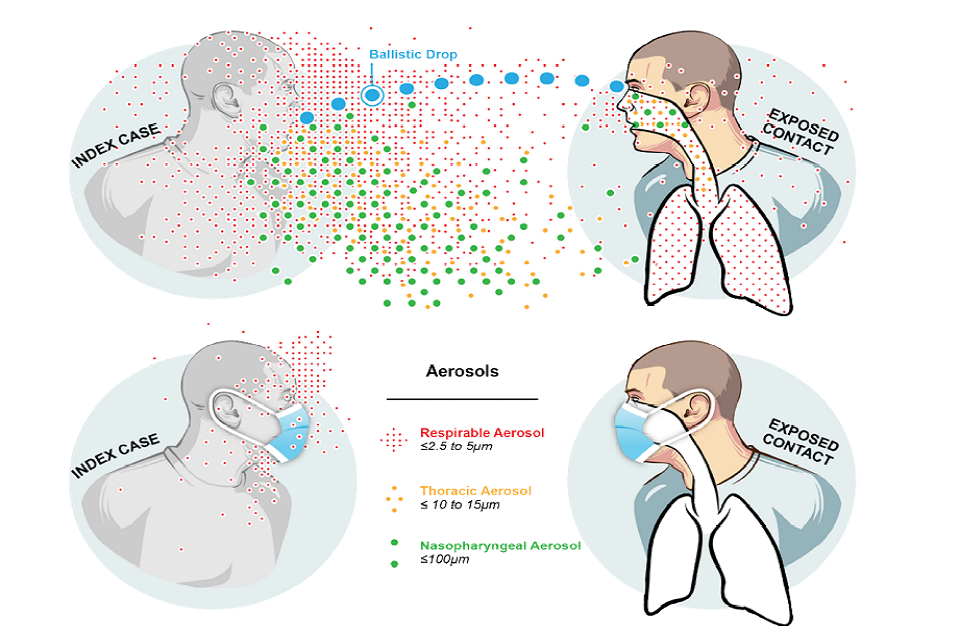
Figure 1: Representative behaviour of different sizes of respiratory particles and the influence of face coverings (reproduced with permission from Milton, 2020)
24. The number of particles released, the range of sizes, and the amount of virus they contain depends on several factors including the viral load of the infected person, their particular respiratory activity (coughing and singing release may release significantly more particles than quiet breathing) and individual characteristics (some people appear to produce far more particles than others) (Morawska et al., 2009; Asadi et al., 2019; Gregson et al., 2020).
25. The amount of SARS-CoV-2 virus contained in different sizes of particles, and whether this changes between people or with activity is not yet known. However, the larger the respiratory particle the more likely it will contain viral particles. The majority of information on the behaviour and size of exhaled microbial particles is generalised from evidence from other microorganisms (Wainwright et al., 2009; Jones-López et al., 2013; Yan et al., 2018; Leung et al., 2020), inert particles or exhalations from healthy volunteers (Xie et al., 2007; Morawska et al., 2009; Davies et al., 2013; Asadi et al., 2019; Gregson et al., 2020)). Some mechanistic evidence uses viral or bacterial challenge aerosols (Bischoff et al., 2011; Lindsley et al., 2014), but only one has used the SARS-CoV-2 virus (Ueki et al., 2020).
26. Early analysis of the B117 variant SARS-CoV-2 virus suggests that it is around 50% to 70% more transmissible (Public Health England, 2020). Analysis from contact tracing data shows that infections for named contacts (secondary attack rate) is 15% for the B117 variant compared to 11% for non-B117 variants (Public Health England, 2020). There is no evidence currently to suggest that the mode of transmission has changed. Emerging evidence for the B117 variant suggests there could be an increased probability of a higher viral load in infected individuals (Kidd et al., 2020). It is also possible that evidence suggesting improved binding to receptors could mean that the amount of virus needed to cause infection is lower (Volz et al., 2021). As previously highlighted (EMG, Dec 2020), for a given exposure there may be a greater likelihood of infection, and this could therefore increase the risks posed by all transmission routes. Recommendations in this paper are based on a precautionary approach, assuming that it is likely that mitigation strategies may now need to be applied more consistently and effectively to achieve a comparable level of control to that seen previously.
27. In the workplace, a context-specific risk assessment is required which considers for each work activity: who is at risk of exposure, how they might be exposed (the relative importance of the different transmission pathways), the likelihood of the people, air or surfaces they are exposed to being infectious, the duration of the exposure, and the consequences of that exposure both in terms of individual risk and population level transmission. A hierarchy of risk controls approach should be applied, and where it is not possible for the activity to be eliminated or a suitable substitute (such as home working) to be applied, then this should consider engineering/environmental, administrative and personal controls to mitigate all transmission routes for all work activities if it is necessary for people to interact.
Application of face coverings
Given the new SARS-Cov-2 variant, what is the role of face coverings in community settings where there is not contact with known infectious individuals, including workplaces?
28. Face coverings and masks can be used for two purposes depending on their design:
a. Source control: this is designed to capture particles that are exhaled by the wearer and acts to reduce the amount of virus that is released into a space. Using a face covering or mask in this way protects multiple other people from the virus that the wearer is emitting
b. Personal protection: this is designed to protect only the person wearing it from being exposed to the virus that is released by someone else
29. Face coverings worn in public, community and workplace settings are predominantly a source control, designed to reduce the emission of virus carrying particles from the mouth and nose of an infected person. This may have measurable benefits in reducing population level transmission when worn widely, through reducing the potential for asymptomatic or pre-symptomatic people spreading the virus without their knowledge. Analysis of regional level data in several countries suggest this impact is typically around 6% to 15% (Cowling and Leung, 2020, Public Health England 2021) but could be as high as 45% (Mitze et al., 2020). These figures should be treated with caution as it is not possible to establish the specific role of face coverings compared to other interventions in place at the same time or how transferable cases from other countries are transferable to the UK. Several modelling studies are reported within a recent systematic review (Howard et al., 2020) that also suggest substantial impacts on the value of R in a population, but are based on idealised assumptions around the efficacy and adherence to wearing face coverings.
30. Public use of face coverings may provide some protection to the wearer, however this is not their intended purpose and there is insufficient evidence to assume that they can provide effective protection when used for this purpose. WHO have recommended that those aged over 60 and those who are vulnerable should wear medical grade face masks (type 2 or 2R) which are likely to provide a more consistent and reliable level of protection than a cloth face covering.
31. The real-world effectiveness of face coverings depends on multiple factors including the materials used, the fit of the face covering and the behaviour of the wearer. Evidence from observational studies evaluated within a recent PHE systematic review (Public Health England, 2021) consistently report that face coverings in the community reduced the spread of COVID-19. However, the PHE review concludes that within ecological studies it is difficult to identify the mechanisms by which face coverings reduce transmission or their effect in isolation from other interventions and behaviours.
32. Mechanistic evidence from laboratory and controlled participant studies suggests most face coverings are likely to be effective at blocking the emission of droplets and larger (nasopharyngeal) aerosol particles but are less effective at limiting the emission of smaller (thoracic and respirable) aerosols. The face covering captures droplets and nasopharyngeal aerosols within the mask material, reducing their emission into the environment. They may also reduce the number of respirable aerosols released, by capturing larger particles before they evaporate (see Annex A). The effectiveness depends on the material and construction of the face coverings, with tighter weave fabrics and multi-layered designs leading to a greater reduction in particle emissions (high confidence). Although there are few studies that directly measure larger particles, many materials will effectively reduce the transport of droplets and nasopharyngeal aerosols. A study with influenza patients showed no deposition onto settle plates held 20 cm from the person due to coughing while wearing medical masks even though deposition was detectable in 7 of 9 participants without masks (Johnson et al., 2009). The reduction in smaller aerosols is typically in the range 50% to 90% depending on the material and construction of the face covering and particle size used for testing (Howard et al., 2020; Lindsley et al., 2020), and is more effective for thoracic aerosols than respirable aerosols (Davies et al., 2013). Laboratory tests have shown that the performance of good quality multi-layer cloth face coverings can be similar to medical grade masks (Davies et al., 2013; Lindsley et al., 2020). However some tests have shown that single layer materials used for face coverings can have filtration efficiencies as low as 5%, and recommend multi-layer construction which is substantially better (Konda et al., 2020). It is likely that this efficiency, especially for smaller aerosols, may be reduced where face coverings are poorly fitting or incorrectly worn.
33. Evidence from visualisation studies suggests that face coverings have an impact on the behaviour of the jets of exhaled air from the nose and mouth, reducing the distance that the jet is maintained and dispersing the exhalation flow around the head of the wearer (Tang et al., 2009; Verma, Dhanak and Frankenfield, 2020). The only study that has measured the close-range impact (Johnson et al., 2009) only considered droplets and didn’t measure microbial aerosols with distance. However it is likely that wearing of a face covering reduces the distance where high concentrations of viral particles are maintained in a respiratory plume, and enables more rapid dilution by the room air.
34. It is possible that wearing a face covering may impact on other behaviours to control the virus such as distancing or hand hygiene. Concerns have been raised that wearing a face covering could decrease other behaviours to reduce transmission. The evidence to date based on observed behaviour does not support this concern with regard to some protective behaviours; neither handwashing nor physical distancing are reduced in those wearing face coverings (Mantzari, Rubin and Marteau, 2020, Czypionka et al., 2020, Seres et al., 2020). Given that face-coverings provide an uncertain level of mitigation or protection it is important that wearing a face covering is not used to justify undertaking or permitting activities and contacts that might otherwise have been avoided. There is some anecdotal evidence that the mandatory use of face coverings may be being used to reduce distancing in some settings.
35. Although contamination of face coverings and subsequent hand contact has been suggested as a transmission route, as highlighted in a previous EMG paper, there is not currently any evidence that this is a significant risk in a community setting (EMG-NERVTAG, 2020). There is some evidence that suggests face coverings may reduce face touching behaviour which could reduce the risk of transmission via contaminated hands (Y. J. Chen et al., 2020). The previous EMG paper (EMG-NERVTAG, 2020) indicated that there are some comfort and hygiene concerns with wearing face coverings, especially for long periods, and recommended that they should be regularly changed (at least every 4 hours) in settings where they may be required for long periods (medium confidence). This previous paper also highlighted the need to regularly wash reusable face coverings, but that there is no clear evidence on frequency or temperature of washing. There is some evidence that cloth face coverings may be more effective after they have been washed as the fibres tighten. It is also possible that washing cloth face coverings may also affect their subsequent fit and thus effectiveness, however studies have not investigated this.
36. There is an absence of evidence that face visors and shields are an effective source control, and are likely to provide a minimal reduction in the source of droplets or aerosols from an infected person. A visualisation study shows that a face visor changes the direction of the exhaled plume, but does not effectively impede it (Verma, Dhanak and Frankenfield, 2020). It is possible that a face visor has some impact on the emission of droplets and nasopharyngeal aerosols through them impacting on the inside of the visor, however this will depend on the direction of the exhalation relative to the angle of the visor. There are several studies that show they can protect against large droplets and splashes (Roberge, 2016) and laboratory evidence from mechanistic studies with an influenza challenge aerosol suggests, that they can provide protection against a source of droplets, nasopharyngeal and thoracic aerosols when someone is in close proximity to an infected person (Lindsley et al., 2014; Nguyen-Van-Tam et al., 2020). It is likely that this includes protection to transmission through the eyes. However they are shown to provide little protection from respirable aerosols (Lindsley et al., 2014; Nguyen-Van-Tam et al., 2020). There does not appear to be any ecological evidence relating to the effectiveness of face visors in mitigating transmission.
Physical Distancing
Given the new SARS-Cov-2 variant, what mitigations are required that may allow less than 2 metres distancing and how they should be implemented, including whether sequencing of mitigations has a role to play in terms of how effective they are.
37. Distancing between people reduces risk of exposure to all viral droplets and aerosols. As you get close to an infected person the likelihood of exposure to all particle sizes increases. Larger particles travel the shortest distances, and so generally pose a risk at close range, while the smallest particles can remain suspended in the air for long periods and can easily travel more than 2 metres in a room. It is important to recognise that there is no guaranteed safe distance within an indoor space – a greater distance for a given exposure time simply reduces the probability of exposure to the virus.
38. At a distance beyond about 2m, exposure to the virus is mainly due to small (respirable and thoracic) aerosols. In an indoor setting these would be dispersed throughout the air in a room, and their concentration is largely determined by the airflow patterns and ventilation rate. Where there is long duration exposure in a poorly ventilated room to someone generating a high number of respirable aerosols, there could be a significant infection risk. The risk of far-field (beyond 2 metres) airborne transmission has been discussed in detail in previous EMG papers (SAGE-EMG, 30 September 2020). There is likely to be a very low risk of exposure to these particles in an outdoor setting as they are quickly dispersed. Modelling suggests that far-field background exposure to the virus in outdoor air is extremely low (Belosi et al., 2021).
39. At a distance of around 1.5 to 2m, exposure to larger (nasopharyngeal) aerosols and droplets is likely to be significantly reduced as these larger particles are more likely to deposit on surfaces within this range. The distance particles are carried before deposition will depend on the force of the respiratory action (a cough or continuous loud talking or singing will project particles further than quiet breathing (Abkarian et al., 2020)), as well as the particular room’s airflow patterns. It is possible that some of these larger particles, which would normally deposit quickly, can be carried further than 2 metres in some cases when released in a turbulent “puff” (Bourouiba, Dehandschoewercker and Bush, 2014) or where there are high velocity airflows. A recent outbreak investigation report suggested high velocities due to an air conditioner may have caused infection in a very short duration exposure (around 5 minutes). The exact mechanisms are uncertain, but the rapid and directional exposure possibly implies larger particles with a high viral concentration may have been carried over a longer distance, rather than people were exposed to smaller aerosols in the general room air (Kwon et al., 2020). There are computational modelling studies that suggest that larger particles may be carried further by wind outdoors (REFS), although there is no evidence of transmission associated with this. The probability of these longer distance exposures through larger particles happening is likely to be low, especially in fluctuating turbulent air in outdoor settings, as it will rely on small numbers of particles being carried over a longer distance to reach a small target area on the face.
40. At a distance less than 2m, without any additional mitigation, exposure to all sizes of respiratory particles will increase as you get closer to a infectious person. The total increase in exposure through all sizes of particles is proportionally greater as the distance decreases; the concentration of respirable and thoracic aerosols is higher close to the infected person, and the risk through exposure to nasopharyngeal aerosols and droplets also increases significantly at the same location. Modelled data (W. Chen et al., 2020) and evidence from an earlier systematic review (Chu et al., 2020) suggests the difference in risk of exposure between 2 metres and 1 metre is likely to be 2 to 10 times, while the difference between 1 metre and 0.5 metres may be as much as 50 times (SAGE-EMG, 2020a, 28 April). These should not be regarded as hard numbers as the complex flows and movement of people in real spaces mean there is significant uncertainty in exposure.
41. The increased transmission risk with reduced distance is also evident to some extent in analysis of UK contact tracing data. The infection rate for reported contacts is almost double for ‘direct’ contact compared to ‘close’ contact as recorded in the contact tracing survey. [footnote 1] Both categories show an increased infection rate with the B117 variant (Public Health England, 2020).
42. The risk of short-range exposure (< 2 metres) is likely to be highest in indoor settings where disruption from room airflows is small, however it could also be important where people have longer duration exposures at close proximity in outdoor settings, especially in semi-enclosed spaces such as covered seating areas, transport shelters or street market stalls. The small amount of evidence for transmission in outdoor settings suggests that it is only likely to be a risk when people are in close interaction (Bulfone et al., 2020). While there is no certainty of benefit in mitigation in these kinds of situations, even minor reductions in transmission at individual level could contribute to meaningful reductions in transmission at population level given a more transmissible virus.
43. The understanding of how respiratory particles behave indicates that additional measures are necessary where people are closer than 2m. This may be due to the role they are doing or where an activity requires it, or where the interactions and behaviour of people in a particular environment means that distancing may be difficult. In a workplace setting the likelihood and frequency of this should be assessed through a context-specific risk assessment. With a more transmissible variant, it is important that previous risk assessments should be revisited and the appropriate mitigations should be implemented and monitored with greater rigour.
44. There is not clear evidence for the relative effectiveness of different mitigations, however interventions that reduce the source of virus, or use engineering or procedural controls that rely less on human behaviour are more likely to have a more reliable impact than those which attempt to use personal behaviour to reduce exposure once the virus is in the environment. It is important that mitigations are targeted at all relevant routes of transmission.
45. Face coverings are likely to be a significant mitigation for exposure at less than 2m. As outlined above, they will impact on risk through reducing the source of respiratory particles. They will mostly reduce the source of droplets and larger aerosols, but may have a small impact on reducing the concentration of smaller aerosols. Their effectiveness will depend on the quality of the face covering and is likely to be strongly influenced by human behaviour.
46. Physical screens and barriers positioned between people are likely to reduce exposure to droplets and larger aerosols, their effect on small (respirable) aerosols depends on the design of the screen and its location relative to the airflow and people in the space. There is very little data on the effectiveness of screens from ecological, modelling or laboratory studies. Evidence from applications on buses suggests that screens which completely separate one person from others in the environment may be effective at reducing their risk of infection providing (UCL, 2020). There remains very little data on the effect of partial screens on transmission risk, with only a small number of experimental (inert tracer) and computational studies prior to and during the pandemic (Ching et al., 2008; Gilkeson et al., 2013; Abuhegazy et al., 2020), and no ecological data to date. These studies highlight that the effect of screens on small aerosols depends significantly on the design relative to room airflows; there may be a reduction in exposure for some designs and an increase for others (Gilkeson et al., 2013; Abuhegazy et al., 2020). Care should be taken when positioning screens that they do not restrict the ventilation in a room; a large screen could separate a space and remove or reduce the ventilation supply for those positioned behind it. Where there is more than one person behind such as screen (for example workers in a shop) this could increase the risk of transmission between these people. Screens are unlikely to have any benefit when people are greater than 2 metre distance, and could increase risks through disruption to airflows.
47. Use of face visors can provide some protection against larger aerosols and droplets as outlined above. This is an approach that focuses on an individual and their exposure, and effectiveness will rely on personal behaviour including correct wearing of the visor.
48. Reducing the duration of any interactions is an important measure which will minimise exposure to all respiratory particles. There is a distribution of risk and exposure will depend on the particular circumstances, however very short exposure (passing in a corridor) will normally pose a much lower risk than spending an extended period of time in close proximity to an infected person. There is not sufficient data to confidently quantify risk of transmission with time of exposure, particularly for the B117 variant.
49. Some benefits may be gained by orienting people to reduce face-to-face interactions, particularly in settings where face coverings are not worn and hence the exhaled air is in the form of a jet from the mouth. Significant care should be used when applying this measure as it is highly dependent on people remaining in a particular location – in many environments people will move around or turn to face others to the side and behind.
50. Deposition of larger aerosols and droplets could increase the contamination of surfaces in close proximity to an infected person. Although there is limited evidence of transmission via contact with contaminated surfaces, paying greater attention to cleaning of high touch surfaces and maintaining awareness of the importance of regular hand hygiene remains important. The use of face coverings is also likely to reduce the deposition of particles on surfaces close to an infected person. However, it is important to recognise that cleaning and hand hygiene alone are not effective mitigations to reduce the risk of direct close-range transmission and any enhanced mitigation should be regarded as a secondary measure alongside other strategies outlined here.
51. Further measures may be needed in a workplace setting where there is a high probability of very close interactions (for example direct physical contact, face to face within 50cm) and it is not possible to remove these or mitigate them in another way. In some workplace settings, bubbling workers and using effective strategies to identify infections early and supporting workers to isolate may reduce the likelihood of transmission. Where there is a significant residual risk which can’t be effectively mitigated it is possible that specific personal protection may be needed, as identified through a suitable risk assessment.
Further Research
- 52. It is clear that there remain several areas where the evidence base is weak or absent, for all variants of the virus including the B117 strain.
- a. There is no clear data yet on the amount of virus exhaled by people and which sizes of particles carry the greatest viral load. This data is very challenging to acquire and requires a substantial human volunteer study with specialist measurement equipment (Leung et al., 2020).
- b. The relative importance of different routes of transmission (close-range, airborne, surface) and how that changes with context is not yet known, although there is growing consensus that direct exposure to respiratory droplets and aerosols through the air is likely to play a greater role than surfaces and fomites (DHS 2021)
- c. The amount of virus needed to cause infection, including whether this changes with infection route or variant of the virus, is unknown (DHS 2021)
- d. There is a lack of data on the absolute and relative effectiveness of different mitigation measures, with a significant absence of data even from idealised laboratory approaches for some approaches (notably screens)
- e. The ability to capture and measure particles larger than 10 to 15 micrometres is very limited, both from a biological and aerosol science perspective. This means that much of the understanding around transmission associated with larger aerosols and droplets is assumed rather than directly measured
Annex A: Emission and behaviour of virus carrying respiratory particles
Respiratory particles are released from the mouth and the nose during breathing, talking, coughing, singing, shouting and sneezing. These particles range from a small number of very large droplets that are up to 1mm in diameter, to large numbers of very small particles that are less than 1 micrometre in diameter. For context, a human hair is around 60 micrometres in thickness and we can’t see particles less than 40 micrometres with the naked eye.
The number and sizes of particles released varies with activity – coughing, sneezing and loud singing or shouting produce up to 20 times more particles than quite talking or just breathing (Gregson et al., 2020). There is a very large variation between people too – some people produce far more particles than others (Morawska et al., 2009; Gregson et al., 2020).
The particles are composed of respiratory fluids containing water, salts, proteins and surfactants as well as virus and other microorganisms (bacteria and fungi depending on the health of the person). Respiratory particles do not remain the same size. When they are released from the moist environment of the mouth and nose into drier air, they rapidly evaporate, losing the water (Xie et al., 2007; Vejerano and Marr, 2018). Their rate of evaporation will depend on the humidity of the air and the constituents of the particles; it is estimated that in a normal room environment, with 50% relative humidity, particles will evaporate to 16% to 50% of their initial size (Nicas, Nazaroff and Hubbard, 2005). As the virus cannot evaporate, this means it can become more concentrated as the particle shrinks. The evaporation for particles smaller than 30 micrometres happens within a fraction of a second. A 100 micrometre particle can evaporate to 30 micrometre particle in 8 seconds, a 50 micrometre particle can become a 10 micrometre particle in under 2 seconds. The evaporation rate will depend on the humidity of the air, with greater evaporation at lower humidity. This is a consideration for UK winter conditions, where in cold weather it is common to have a relative humidity below 35% in indoor environments. It is possible that this would result in a greater proportion of particles evaporating to a size where they can remain airborne. There is also evidence that the SARS-CoV-2 virus, in common with many other viruses, survives better at lower humidity.
There is some confusion caused by terminology surrounding respiratory particles. Medical fields use the term “airborne” to refer to infection via particles with a final size less than 5 micrometres in diameter and refer to infection via all other particle sizes as droplets. Aerosol scientists refer to particles with a final size up to 100 micrometres as aerosols, which is based on physics of particle transport and exposure science evidence showing these can be inhaled into the upper respiratory system. The term aerosol in medicine often refers specific procedures (aerosol generating procedures) that release very small particles < 10 micrometres. Care needs to be taken as terms are used differently in different contexts. A good discussion of these particles is given in ‘Milton (2020)’ which suggests four categories of particles that can be used to describe both their fate in air and the exposure mechanism:
- respirable aerosols are < 5 micrometres, will remain airborne for long periods and can penetrate to the deep lung on inhalation
- thoracic aerosols are 5 to 15 micrometres, will remain airborne in many rooms over more than 2 metres and will deposit in the thorax on inhalation
- nasopharyngeal aerosols are 15 to 100 micrometres, will normally only remain airborne for 1 metre to 2 metres unless velocities are high, and will deposit in the nasal cavities and mouth following inhalation
- droplets are particles >100 micrometres, behave ballistically depositing normally within 2 metres and can cause infection by direct deposition onto mucous membranes
The amount of virus released in respiratory particles is likely to increase with the viral load of the infected person (which is highest around the time of symptom onset), increase with greater levels of vocal activity, and will vary by person. There is very little data yet for SARS-CoV-2, and none for the B117 variant, however evidence for other viruses suggests this is the case (Yan et al., 2018). Data from nasal swabs suggest the amount of virus RNA per ml of respiratory fluid could vary enormously between 102 and 109 (Pan et al., 2020). The amount of virus carried by different particle sizes is not certain. It is estimated that larger particles will contain more virus due to their larger volume, however there is data from exhaled breath samples with other pathogens (Wainwright et al., 2009; Jones-López et al., 2013; Yan et al., 2018; Leung et al., 2020) that suggests there could be proportionally more pathogens in smaller particles. However this may reflect the inability of commonly used samplers to effectively sample particles > 10 micrometres diameter This is in part likely to be due to the effect of evaporation which concentrates the virus in smaller particles, however it could be also affected by the location in the respiratory system where the particle is formed and for some microorganisms there are hydrophobic effects that can lead to preferential aerosolization into smaller particles. It is not clear how SARS-COV-2 behaves yet and measurement of microorganisms in exhaled breath is extremely challenging.
The fate of a particle in air depends on its size and the airflow in the space where it is released. Droplets that remain greater than 100 micrometres in diameter, typically behave ballistically and fly through the air with a trajectory that is determined by their size, gravity and the force of the ejection – think how a tennis ball behaves. These particles typically land on surfaces or another person within 2m, but could be carried further in high velocity airflows, particularly outdoors. To cause infection these particles would impact directly on eyes, nose or mouth or they would contaminate a surface and create a transmission route via hand contact. The number of these larger particles generated by people is small (Chao et al., 2009; Morawska et al., 2009) and hence the chance of infection depends on the probability that they hit a susceptible target – this is likely to be a rare event, but could carry a high likelihood of infection if it happens.
The fate of particles that are smaller than 100 micrometres will depend on their evaporation. Those that have a final size greater than 10 micrometres (nasopharyngeal and some thoracic aerosols) will normally deposit out of the air relatively quickly. This is often within 2 metres of the source, but it is possible that some can be carried further by the complex fluid dynamics of a turbulent “puff” released from the mouth (Bourouiba, 2020), or by higher velocity flows. There is some evidence that air conditioning units may create flows that can maintain particles that are 20 to 30 micrometres airborne over greater distances (Kwon et al., 2020; Lu et al., 2020). When carrying virus, these particles can cause infection by inhalation; they will land in the upper part of the respiratory tract, but they don’t reach the lung. While this is not important for some airborne diseases where the infection route is via the lung (for example tuberculosis, TB), it is likely to be important for SARS-CoV-2 where the virus binds to ACE2 receptors that are throughout the respiratory system including in the eyes, mouth and nose. Relatively large numbers of particles that become nasopharyngeal aerosols are generated through respiratory activities, and as they may carry a high viral load they are likely to pose a large risk of transmission if someone is exposed at close-range.
Particles with a final size less than 10 micrometres (respirable and some thoracic aerosols) can remain suspended in the air indoors for long periods of time as their settling speed is usually lower than the buoyant forces and room airflow patterns that keep them suspended in air. While exposure in air is the primary concern with particles in these size ranges, it should be noted that deposition does occur, even at particles smaller than 2 micrometres which can contaminate surfaces (King et al., 2013). These particles are at highest concentration close to the infected person, especially directly in their exhaled breath, and reduce in concentration with distance. By 1.5 to 2 metres the concentration of these particles in a room is determined by the ventilation – a poorly ventilated room will not remove the particles as quickly. These particles cause infection by inhalation, and the smallest ones (< 5 micrometres) can penetrate to the deep lung. They are the main transmission route for diseases like TB, however could also be important for viruses such as influenza and SARS-CoV-2. Large numbers of these particles are generated by respiratory activities (Chao et al., 2009; Morawska et al., 2009; Asadi et al., 2019), however as they carry a lower viral load than larger aerosols and droplets, it may be necessary to inhale a greater number to enable transmission of infection. High concentrations close to an infected person or following long duration exposure in a poorly ventilated space may enable these particles to pose a risk.
References
Abkarian, M. et al. (2020) ‘Speech can produce jet-like transport relevant to asymptomatic spreading of virus’, ‘Proceedings of the National Academy of Sciences of the United States of America’, 117(41), pp.25237–25245. doi: 10.1073/pnas.2012156117.
Abuhegazy, M. et al. (2020) ‘Numerical investigation of aerosol transport in a classroom with relevance to COVID-19’, ‘Physics of Fluids’. AIP Publishing LLC, 32(10). doi: 10.1063/5.0029118.
Asadi, S. et al. (2019) ‘Aerosol emission and superemission during human speech increase with voice loudness’, ‘Scientific Reports’. Springer US, 9(1), pp. 1–10. doi: 10.1038/s41598-019-38808-z.
Belosi, F. et al. (2021) ‘On the concentration of SARS-CoV-2 in outdoor air and the interaction with preexisting atmospheric particles’, ‘Environmental Research’. Elsevier Inc., 193(November 2020), p. 110603. doi: 10.1016/j.envres.2020.110603.
Bischoff, W. E. et al. (2011) ‘Transocular entry of seasonal influenza-attenuated virus aerosols and the efficacy of N95 respirators, surgical masks, and eye protection in humans’, Journal of Infectious Diseases, 204(2), pp. 193–199. doi: 10.1093/infdis/jir238.
Bourouiba, L. (2020) ‘Turbulent Gas Clouds and Respiratory Pathogen Emissions: Potential Implications for Reducing Transmission of COVID-19’, ‘JAMA - Journal of the American Medical Association’, pp. E1–E2. doi: 10.1001/jama.2020.4756.
Bourouiba, L., Dehandschoewercker, E. and Bush, J. W. M. (2014) ‘Violent expiratory events: On coughing and sneezing’, ‘Journal of Fluid Mechanics’, 745, pp. 537–563. doi: 10.1017/jfm.2014.88.
BSI 2020, BSI Kitemark Certification for face coverings
Bulfone, T. C. et al. (2020) ‘Outdoor Transmission of SARS-CoV-2 and Other Respiratory Viruses, a Systematic Review’, ‘The Journal of Infectious Diseases’, pp. 1–44. doi: 10.1093/infdis/jiaa742.
Chao, C. Y. H. et al. (2009) ‘Characterization of expiration air jets and droplet size distributions immediately at the mouth opening’, ‘Journal of Aerosol Science’, 40(2), pp. 122–133. doi: 10.1016/j.jaerosci.2008.10.003.
Chen, W. et al. (2020) ‘Short-range airborne route dominates exposure of respiratory infection during close contact’, ‘Building and Environment’. Elsevier Ltd, pp. 1–33. doi: 10.1016/j.buildenv.2020.106859.
Chen, Y. J. et al. (2020) ‘Comparison of Face-Touching Behaviors Before and During the Coronavirus Disease 2019 Pandemic’, ‘JAMA network open’, 3(7), p. e2016924. doi: 10.1001/jamanetworkopen.2020.16924.
Ching, W. H. et al. (2008) ‘Reducing risk of airborne transmitted infection in hospitals by use of hospital curtains’, ‘Indoor and Built Environment’, 17(3), pp. 252–259. doi: 10.1177/1420326X08091957.
Chu, D. K. et al. (2020) ‘Articles Physical distancing, face masks, and eye protection to prevent person-to-person transmission of SARS-CoV-2 and COVID-19: a systematic review and meta-analysis’, 6736(20).
Czypionka T, Greenhalgh T, Bassler D, Bryant MB. Masks and face coverings for the lay public: A narrative update. Annals of internal medicine. 2020 October 2020.
Cowling, B. J. and Leung, G. M. (2020) ‘Face masks and COVID-19: don’t let perfect be the enemy of good’, ‘Euro surveillance : bulletin Europeen sur les maladies transmissibles = European communicable disease bulletin’. European Centre for Disease Prevention and Control (ECDC), 25(49), pp. 1–4. doi: 10.2807/1560-7917.ES.2020.25.49.2001998.
Davies, A. et al. (2013) ‘Testing the efficacy of homemade masks: would they protect in an influenza pandemic?’, ‘Disaster medicine and public health preparedness’, 7(4), pp. 413–418. doi: 10.1017/dmp.2013.43.
DHS Master List, update 5 January 2021.
EMG-NERVTAG (2020) ‘Duration of Wearing of Face Coverings’, pp. 2–7.
EMG, S. (2020) ‘Mitigations to Reduce Transmission of the new variant SARS-CoV-2 virus’, December 31 2020.
Gilkeson, C. A. et al. (2013) ‘Measurement of ventilation and airborne infection risk in large naturally ventilated hospital wards’, ‘Building and Environment’. Elsevier Ltd, 65, pp. 35–48. doi: 10.1016/j.buildenv.2013.03.006.
Gregson, F. K. A. et al. (2020) ‘Comparing the Respirable Aerosol Concentrations and Particle Size Distributions Generated by Singing, Speaking and Breathing’, ‘ChemRxiv’, (1), pp. 1–27. doi: 10.26434/chemrxiv.12789221.v1.
Howard, J. et al. (2020) ‘Face masks against COVID-19: An evidence review’, 118(4), pp. 1–12. doi: 10.1073/pnas.2014564118.
Johnson, D. F. et al. (2009) ‘A quantitative assessment of the efficacy of surgical and N95 masks to filter influenza virus in patients with acute influenza infection’, ‘Clinical Infectious Diseases’, 49(2), pp. 275–277, doi: 10.1086/600041.
Jones-López, E. C. et al. (2013) ‘Cough aerosols of Mycobacterium tuberculosis predict new infection: A household contact study’, ‘American Journal of Respiratory and Critical Care Medicine’, 187(9), pp. 1007–1015. doi: 10.1164/rccm.201208-1422OC.
Kidd, M. et al. (2020) ‘S-variant SARS-CoV-2 is associated with significantly higher viral loads in samples tested by ThermoFisher TaqPath RT-QPCR.’, medRxiv.
King, M. F. et al. (2013) ‘Bioaerosol deposition in single and two-bed hospital rooms: A numerical and experimental study’, ‘Building and Environment’, 59, pp. 436–447. doi: 10.1016/j.buildenv.2012.09.011.
Konda, A. et al. (2020) ‘Fabrics Used in Respiratory Cloth Masks’.
Kwon, K. S. et al. (2020) ‘Evidence of Long-Distance Droplet Transmission of SARS-CoV-2 by Direct Air Flow in a Restaurant in Korea’, ‘Journal of Korean Medical Science’, 35(46), pp. 1–8. doi: 10.3346/JKMS.2020.35.E415.
Leung, N. H. L. et al. (2020) ‘Respiratory virus shedding in exhaled breath and efficacy of face masks’, ‘Nature Medicine 2020’. Springer US, pp. 1–5. doi: 10.1038/s41591-020-0843-2.
Lindsley,W. G. et al. (2014) ‘Efficacy of face shields against cough aerosol droplets from a cough simulator’, ‘Journal of Occupational and Environmental Hygiene’, 11(8), pp. 509–518. doi: 10.1080/15459624.2013.877591.
Lindsley, W. G. et al. (2020) ‘Efficacy of face masks, neck gaiters and face shields for reducing the expulsion of simulated cough-generated aerosols’, ‘Aerosol Science and Technology’. Taylor & Francis, 0(0), pp. 1–12. doi: 10.1080/02786826.2020.1862409.
Lu, J. et al. (2020) ‘COVID-19 Outbreak Associated with Air Conditioning in Restaurant, Guangzhou, China, 2020’, ‘Emerging infectious diseases’, 26(7), pp. 1628–1631. doi: 10.3201/eid2607.200764.
Mantzari, E., Rubin, G. J. and Marteau, T. M. (2020) ‘Is risk compensation threatening public health in the COVID-19 pandemic?’, BMJ (Clinical research ed.), 370, p. m2913. doi: 10.1136/bmj.m2913.
Milton, D. K. (2020) ‘A Rosetta Stone for Understanding Infectious Drops and Aerosols’, Journal of the Pediatric Infectious Diseases Society, 9(4), pp. 413–415. doi: 10.1093/jpids/piaa079.
Mitze, T. et al. (2020) ‘Face masks considerably reduce COVID-19 cases in Germany’, Proceedings of the National Academy of Sciences of the United States of America, 117(51), pp. 32293–32301. doi: 10.1073/pnas.2015954117.
Morawska, L. et al. (2009) ‘Size distribution and sites of origin of droplets expelled from the human respiratory tract during expiratory activities’, ‘Journal of Aerosol Science’, 40(3), pp. 256–269. doi: 10.1016/j.jaerosci.2008.11.002.
Nguyen-Van-Tam, J. S. et al. (2020) ‘Minimal transmission in an influenza A (H3N2) human challenge transmission model within a controlled exposure environment’, PLoS Pathogens, 16(7), pp. 1–16. doi: 10.1371/journal.ppat.1008704.
Nicas, M., Nazaroff, W. W. and Hubbard, A. (2005) ‘Toward understanding the risk of secondary airborne infection: Emission of respirable pathogens’, ‘Journal of Occupational and Environmental Hygiene’, 2(3), pp. 143–154. doi: 10.1080/15459620590918466.
Pan, Y. et al. (2020) ‘Viral load of SARS-CoV-2 in clinical samples’, ‘The Lancet Infectious Diseases’. Elsevier Ltd, 20(4), pp. 411–412. doi: 10.1016/S1473-3099(20)30113-4.
Public Health England (2020) ‘Investigation of novel SARS-COV-2 variant Variant of Concern 202012 / 01 Detection of an epidemiological cluster associated with a new variant of concern Nomenclature of variants in the UK Current epidemiological findings’, (December), pp. 1–11.
Roberge, R. J. (2016) ‘Face shields for infection control: A review’, ‘Journal of Occupational and Environmental Hygiene’. Taylor & Francis, 13(4), pp. 239–246. doi: 10.1080/15459624.2015.1095302.
SAGE-EMG (2020a) ‘Environmental Influence on Transmission’, 28 April 2020.
SAGE-EMG (2020b) ‘Role of Ventilation in Controlling SARS-CoV-2 Transmission’, 30 September 2020, pp. 2–33.
Seres G, Balleyer A, Cerutti N, Danilov A, Friedrichsen J, Liu Y, Süer M. Face Masks Increase Compliance with Physical Distancing Recommendations During the COVID-19 Pandemic. argument. 2020 May 23;20:44.
Seres G, Balleyer AH, Cerutti N, Friedrichsen J. Face mask use and physical distancing before and after mandatory masking: Evidence from public waiting lines. Available at SSRN 3641367. 2 July 2020.
Tang, J. W. et al. (2009) ‘A schlieren optical study of the human cough with and without wearing masks for aerosol infection control’, ‘Journal of the Royal Society Interface’, 6(SUPPL. 6), pp. 727–736. doi: 10.1098/rsif.2009.0295.focus.
Ueki, H. et al. (2020) ‘Effectiveness of Face Masks in Preventing Airborne Transmission of SARS-CoV-2’, mSphere, 5(5), pp. 2–6. doi: 10.1128/msphere.00637-20.
Vejerano, E. P. and Marr, L. C. (2018) ‘Physico-chemical characteristics of evaporating respiratory fluid droplets’, ‘Journal of the Royal Society Interface’, 15(139), pp. 1–10. doi: 10.1098/rsif.2017.0939.
Verma, S., Dhanak, M. and Frankenfield, J. (2020) ‘Visualizing the effectiveness of face masks in obstructing respiratory jets’, ‘Physics of Fluids’, 32(6). doi: 10.1063/5.0016018.
Volz, E. et al. (2021) ‘Transmission of SARS-CoV-2 Lineage B .1 .1 .7 in England : Insights from linking epidemiological and genetic data’.
Wainwright, C. E. et al. (2009) ‘Cough-generated aerosols of Pseudomonas aeruginosa and other Gramnegative bacteria from patients with cystic fibrosis’, ‘Thorax’, 64(11), pp. 926–931. doi: 10.1136/thx.2008.112466.
UCL 2020, Covering screen gaps minimises COVID-19 risk for bus drivers
Xie, X. et al. (2007) ‘How far droplets can move in indoor environments - revisiting the Wells evaporation- falling curve’, ‘Indoor Air’, 17(3), pp. 211–225. doi: 10.1111/j.1600-0668.2007.00469.x.
Yan, J. et al. (2018) ‘Infectious virus in exhaled breath of symptomatic seasonal influenza cases from a college community’, ‘Proceedings of the National Academy of Sciences of the United States of America’, 115(5), pp. 1081–1086. doi: 10.1073/pnas.1716561115.
End notes
-
Direct: face to face contact (for example a conversation within one metre); skin to skin contact (including sexual contact); coughed on, sneezed on or spat on.
Close: within 1 metre for 1 minute or more (not necessarily face to face); within 1 to 2 metres for 15 minutes or more (could be total 15 minutes over 24 hours); travelling in a small vehicle; travelling in a large vehicle or plane (one metre for one minute and 1 to 2 metres for 15 minutes). ↩